Combination of External Beam Radiation Therapy and Immune Checkpoint Inhibitors in Cancer Treatment: Mechanisms, Limitations, an
Affiliations
- 1 Department of Cancer Biology, University of Wisconsin-Madison, Madison WI
- 2 Department of Medical Physics, University of Wisconsin-Madison, Madison WI
- 3 Department of Human Oncology, University of Wisconsin-Madison, Madison WI
- 4 Carbone Cancer Center, University of Wisconsin-Madison, Madison WI
- 5 William S. Middleton Memorial Veterans Hospital, Madison WI
External beam radiation therapy (EBRT) has long been integral in cancer treatment, effectively targeting localized and metastatic tumors. Immunotherapy, especially immune checkpoint inhibitors (ICIs), leverages the immune system to eliminate cancer cells but faces challenges such as treatment resistance. EBRT may provide an approach to overcoming resistance to ICI therapy, thus enhancing ICIs’ efficacy and broadening their clinical scope. EBRT, by inducing immunogenic cell death, primes the immune system and can potentiate ICIs. This combination strategy has shown promise in preclinical studies, highlighting the potential of EBRT to overcome the limitations of ICI monotherapy and vice versa. Clinical trials have demonstrated the safety and feasibility of this combination, with evidence suggesting improved tumor control and patient outcomes. Nevertheless, numerous challenges remain. This review explores the mechanisms, challenges, and clinical trials evaluating the combination of EBRT and ICIs, underscoring the need for optimized approaches to maximize clinical efficacy, while minimizing toxicities.
Introduction
Radiation therapy (RT) is a pillar in cancer therapy, predominantly delivered in the clinical setting by linear accelerators as external beam radiation therapy (EBRT) to eradicate cancer cells or provide symptom relief.1 By inducing DNA damage in cancer cells, RT disrupts their ability to divide and proliferate, ultimately leading to cell death.2 Over the years, RT has evolved significantly with advances in both technology and methodology, enhancing its precision while minimizing damage to surrounding healthy tissues.3
The integration of advanced imaging and computer technologies has profoundly transformed RT planning and delivery, significantly enhancing treatment safety and patient outcomes.4
Intensity-modulated radiation therapy, image-guided radiation therapy, and stereotactic body radiation therapy (SBRT) represent major technological advances that have made EBRT an effective and indispensable tool in modern oncology.5, 6
Exploring the role of RT in enhancing the effectiveness of immune checkpoint blockade (ICB) therapy has gained attention as a promising strategy in advancing cancer treatment. In recent years, immunotherapy has gained considerable clinical attention, with ICB emerging as a transformative strategy in cancer therapy.7 ICB therapy with immune checkpoint inhibitors (ICIs) targets immune checkpoints, such as CTLA-4 and PD-1/PD-L1, which tumors exploit to suppress T-cell activity.8, 9 By suppressing the inhibition signal from these immune checkpoints, ICB boosts the immune system, leading to durable tumor regression and improved survival outcomes in cancers, including melanoma, non-small cell lung cancer, and renal cell carcinoma.10
Resistance to ICB Therapy
Patients receiving ICIs as monotherapy can develop primary resistance, and thus never respond to ICIs, or acquire resistance and subsequently develop disease progression after an initial response. While there are numerous mechanisms underlying the resistance to ICB, they are broadly dichotomized into tumor-intrinsic or tumor-extrinsic factors. Tumor-intrinsic mechanisms include the loss of neoantigens, especially in low-tumor mutational burden disease, aberrations in cell signaling and metabolic pathways, loss of major histocompatibility complex (MHC) I expression resulting in decreased antigen presentation, and epigenetic gene silencing through DNA demethylation and histone deacetylation. Tumor-extrinsic mechanisms encompass factors such as a decrease in immune cell infiltration in the tumor microenvironment (TME), compensatory upregulation of other immune checkpoint molecules, epithelial-mesenchymal transition, and aberration in angiogenesis. For further reading, Alsaafeen et al provide a comprehensive discussion of the mechanisms of resistance to ICB.11
Radiation to Enhance the Efficacy of ICB
Aside from directly eliminating cancer cells, EBRT also possesses immunomodulatory effects. A key mechanism of such immunomodulation is the activation of type I interferon (IFN1) response through the cyclic GMP-AMP (cGAMP) synthase and stimulator of interferon genes (cGAS-STING) pathway.12 - 15 This results in the production of IFNβ, which promotes the activation of dendritic cells and tumor antigen-presenting cells, leading to T-cell activation and an antitumor immune response.15, 16 In preclinical models, EBRT-induced IFN1 responses have been shown to convert immunologically “cold” tumors, lacking immune cell infiltration into the TME, into immunologically “hot” tumors.17, 18 This shift subsequently boosts the immune response that can be further potentiated by cytokines secreted by irradiated tumor cells . 15, 19 Additionally, post-RT immune modulation activates CD8+ T cells, increasing the number of stem-like CD8+ T cells, which become terminally differentiated effector cells responsible for tumor destruction. Tumor-draining lymph nodes (LNs) serve as reservoirs for these stem-like CD8+ T cells, facilitating their expansion and migration to the tumor. Interestingly, targeting both the LN and tumor with RT reduces the abscopal effect and decreases the number of tumor-specific and stem-like CD8+ T cells, highlighting the important role of LNs in mediating the abscopal response.20 RT also induces the release of exosomes from tumor cells capable of stimulating dendritic cell maturation and promoting natural killer (NK) cell infiltration into the TME. This immune activation significantly delays tumor growth, with NK cells producing IFNγ as a key mediator of such antitumor response. The subsequent depletion of NK cells abolishes this effect, underscoring their pivotal role in the immune response.21 As such, the aforementioned immunostimulatory effects of EBRT can be exploited to enhance suboptimal clinical efficacy of ICIs.
Combining EBRT With ICIs in Cancer Treatment: Rationale and Preclinical Data
The combination of EBRT and ICIs represents a promising frontier in cancer treatment, with the capacity to enhance patient outcomes through synergistic mechanisms. This dual approach leverages radiation’s ability to enhance tumor immunogenicity by triggering the release of tumor antigens and damage-associated molecular patterns, such as calreticulin and high mobility group box 1 (HMGB1).22 These effects can create an “in situ vaccine,” effectively priming immune cells to recognize and attack the tumor, thereby enhancing the overall immune response.23 EBRT also increases the expression of tumor-associated antigens and MHC molecules, further making tumors more susceptible to immune recognition and eradication.24 Radiation also induces the expression by the tumor of neoantigens, stimulating the expansion of CD8+ T cells, potentially contributing to an abscopal response.25 This combination approach has also been found to increase the infiltration of cytotoxic T lymphocytes into the TME and the release of proinflammatory cytokines, potentiating the immune response.26 - 29 Figure 1 summarizes the potential synergistic interactions between radiation delivered by EBRT and ICIs.
Synergistic effects between radiation therapy (RT) and immunotherapy (IT) in improving tumor control. Red arrows highlight the mechanisms by which RT enhances the immune response facilitated by IT, while green arrows depict how IT strengthens the therapeutic outcomes of RT.
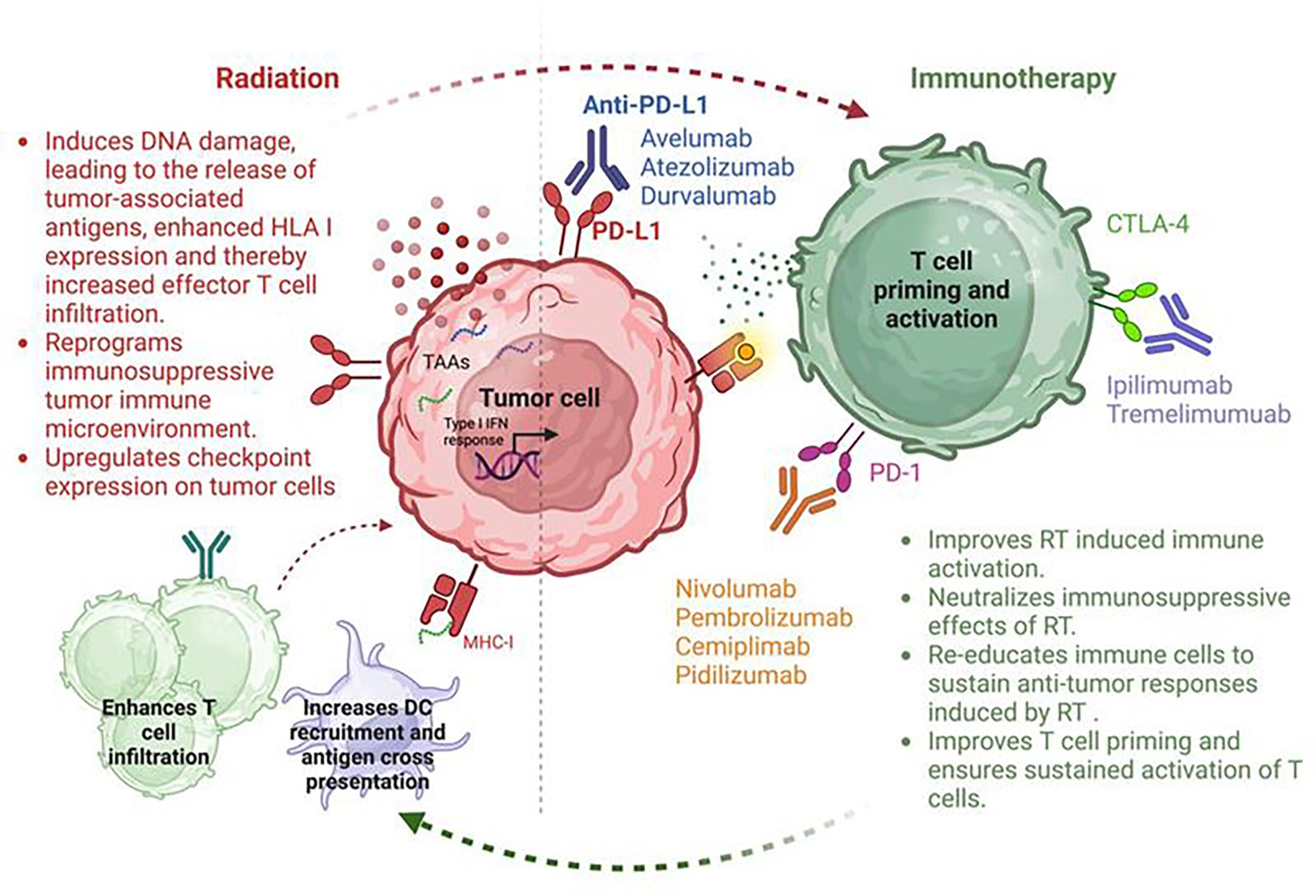
Several preclinical studies have explored the potential of combining EBRT with ICIs. Verbrugge et al demonstrated that concurrent radiation and PD-1 blockade enhanced the curative effects of radiation in a murine breast cancer model.30 Sharabi and colleagues showed that SBRT, given 1 day prior to PD-1 blockade, enhanced the antitumor immune response and led to the formation of memory T cells through cross-presentation of tumor antigens.31 Furthermore, Friedman et al showed that response to SBRT can be augmented by concurrent treatment with anti-PD-1.32
Despite the promising results of combining RT with ICB, determining the optimal approach for this combination remains an area of active research. Key factors such as radiation dose, fractionation schemes, and treatment sequencing continue to be explored to maximize the therapeutic benefits.33
Clinical Trials Investigating the Combination of EBRT and ICIs
Combining EBRT with ICIs has emerged as a promising approach to enhance antitumor immune responses and improve patient outcomes across multiple cancer types as shown in Table 1 . Herein, we focus our discussion mostly on phase III trials.
Selected Clinical Trials Evaluating Radiation Therapy Combined With Immune Checkpoint Blockade in Cancer
Cancer Type | Trial Identifier | Phase | Disease Stage | RT Type | RT Dose/ Fractionation | Combination Immunotherapy | Immunotherapy Dose | Timing | Trial Status/Result | Reference |
---|---|---|---|---|---|---|---|---|---|---|
NSCLC | NCT02434081 (PACIFIC trial) | III | Stage III NSCLC | EBRT | 60-66 Gy in 30-33 fractions | Durvalumab | 10 mg/kg every 2 wk | Sequential | Completed; improved PFS and OS | 34 |
NCT02788404 (PACIFIC 2 trial) | III | Stage III NSCLC | EBRT | 60-66 Gy in 30-33 fractions | Durvalumab | 1500 mg every 4 wk | Concurrent | Completed; no improvement in PFS and OS | 35 | |
NCT02492568 (Pembro-RT) | II | Metastatic NSCLC | SBRT | 27 Gy in 3 fractions | Pembrolizumab | 200 mg/kg every 3 wk | Sequential | Completed; trend toward improved PFS and OS | 36 | |
NCT03110978 | II | Early stage NSCLC | SABR | 50 Gy in 4 fractions or 70 Gy in 10 fractions | Nivolumab | 480 mg every 4 wk | Concurrent | Completed; significantly improved 4-y event-free survival | 37 | |
SCLC | NCT02046733 (STIMULI trial) | II | Limited-stage SCLC | EBRT | Recommended: 45 Gy twice daily Allowed: 56 Gy once daily | Nivolumab + ipilimumab | Nivolumab (1 mg/kg) + ipilimumab (3 mg/kg) every 3 wk→ nivolumab (240 mg) every 2 wk | Sequential | Completed; no improvement in PFS | 38 |
NCT03703297 (ADRIATIC trial) | III | Limited-stage SCLC | EBRT | 60-66 Gy once daily or 45 Gy twice daily | Durvalumab alone or in combination with tremelimumab | Durvalumab alone (1500 mg) every 4 wk or durvalumab (1500 mg)+ tremelimumab (75 mg) every 4 wk | Sequential | Ongoing; interim results: improvement of OS with durvalumab | 39 | |
Head and neck cancer | NCT02952586 (JAVELIN Head & Neck 100) | III | Locally advanced HNSCC | EBRT | 70 Gy in 35 fractions | Avelumab | 10 mg/kg every 2 wk | Sequential | Completed; no PFS and OS improvement | 40 |
NCT03452137 (IMvoke010 trial) | III | High-risk locally advanced HNSCC | EBRT | Definitive treatment (surgery or CRT) | Atezolizumab | 1200 mg every 3 wk | Sequential | Terminated; no event-free survival (EFS) or OS improvement | 41 | |
NCT03811015(ECOG ACRIN EA3161) | II/III | Locally advanced Human papillomavirus (HPV) + oropharyngeal carcinoma | EBRT | 70 Gy in 35 fractions | Nivolumab | Every 4 wk | Sequential | Ongoing | 42 | |
Gastrointestinal (GI) cancers | NCT03604991 (ECOG-ACRIN EA2174) | II/III | Locoregional esophageal and gastroesophageal junction (GEJ) adenocarcinoma | EBRT | 41.4-50.4 Gy in 1.8 Gy /fraction | Nivolumab + ipilimumab | ---- | Perioperative | Ongoing | 43 |
NCT04210115 (KEYNOTE-975) | III | Unresectable esophageal carcinoma | EBRT | 50 or 60 Gy | Pembrolizumab | 200 mg every 3 wk (8 cycles) → 400 mg every 6 wk (5 cycles) | Concurrent→ adjuvant | Ongoing | 44 | |
Genitourinary (GU) cancers | NCT00861614 | III | Metastatic castration-resistant prostate cancer | EBRT | 8 Gy in one fraction | Ipilimumab | 10 mg/kg | Sequential | Completed; no statistically significant OS but survival benefit for patients with favorable prognostic factors (no visceral mets or anemia) | 45 |
NCT04241185 (KEYNOTE-992) | III | Muscle-invasive bladder | EBRT | 64 Gy in 2 Gy/ fraction or 55 Gy in 2.75 Gy/ fraction | Pembrolizumab | 400 mg every 6 wk | Concurrent→ adjuvant | Ongoing | 46 | |
Cervical cancers | NCT04221945 (ENGOT-cx11/GOG-3047/KEYNOTE-A18) | III | High-risk locally advanced cervical cancer | EBRT ±bracytherapy | Median total cervix dose 76 Gy (median total EQD 2Gy: 87 Gy) | Pembrolizumab | 200 mg every 3 wk + CRT → 400 mg every 6 wk (15 cycles) | Concurrent → adjuvant | Improvement of PFS | 47 |
Abbreviations: CRT, chemoradiotherapy; EBRT, external beam radiation therapy; EFS, event-free survival; HNSCC, head and neck squamous cell carcinoma; HPV, human papillomavirus; GEJ, gastroesophageal junction; GEJ, gastroesophageal junction; GI, gastrointestinal; GU, genitourinary; NSCLC, non-small cell lung cancer; OS, overall survival; PFS, progression-free survival; RT, radiation therapy; SABR, stereotactic ablative body radiation therapy; SCLC, small cell lung cancer; SBRT, stereotactic body radiation therapy.
Non-Small Cell Lung Cancer (NSCLC)
The PACIFIC trial remains the cornerstone study for combining immunotherapy with EBRT in NSCLC.48 This phase III trial showed that compared with placebo, durvalumab, administered sequentially 1 to 42 days after chemoradiotherapy (CRT) significantly improved progression-free survival (PFS) (median: 16.9 vs 5.6 mo) and overall survival (OS) (median: 47.5 vs 29.1 mo) in patients with unresectable stage III NSCLC.34 Thus, it cemented the role of durvalumab in the management of unresectable stage III NSCLC.
Considering the success of the PACIFIC trial, the PACIFIC 2 phase III trial evaluated the concurrent administration of durvalumab vs placebo with CRT followed by consolidation with durvalumab or placebo in patients with unresectable stage III NSCLC.49 Unfortunately, no statistically significant improvement in the PFS (HR, .85; 95% CI: .65-1.12; P = .247) or OS (HR, 1.03; 95% CI: .78-1.39; P = .823) was noted.35 The observed difference between the outcomes of the PACIFIC and PACIFIC 2 trials highlights the crucial role of the sequencing of the combination and suggests that with standard fractionation, sequential combination of durvalumab with CRT in patients with unresectable stage III NSCLC may be superior to a concurrent administration.
With respect to ablative radiation dose regimen, in the metastatic setting the PEMBRO-RT phase II trial reported a doubling of the objective response rate (ORR) with pembrolizumab administered after SBRT (24 Gy in 3 fractions), 36% compared with 18% with pembrolizumab alone. Although trends toward improvement of the median PFS (6.6 vs 1.6 mo) and median OS (15.9 vs 7.6 mo) were noted with pembrolizumab plus SBRT, these were not statistically significant due to the small sample size of the study cohort.36 In early stage disease, a randomized phase II trial (I-SABR) by Chang et al demonstrated a significant improvement of the 4-year event-free survival with the combination of stereotactic-ablative radiation therapy (SABR) and 4 cycles of nivolumab (77%) compared with SABR alone (53%).37
Small Cell Lung Cancer (SCLC)
The STIMULI phase II trial evaluated the consolidation immunotherapy with ipilimumab and nivolumab compared with observation after CRT in limited-stage (LS) SCLC. No improvement in the PFS was noted, and high toxicity rates dampened the efficacy of this therapeutic combination.38 The ADRIATIC phase III trial randomized patients with LS SCLC to receive after CRT durvalumab alone, durvalumab plus tremelimumab, or placebo. Interim results revealed that adjuvant durvalumab led to a significant improvement of OS compared with placebo (median OS: 55.9 mo, 95% CI: 37.3-not reached; vs 33.4 mo, 95% CI: 25.5-39.9; HR: .73, 98% CI: .54-.98; P = .01). Although the rates of grade 3 or 4 toxicities were similar in patients receiving durvalumab or placebo, 24.4% and 24.2%, respectively, treatment stoppage was higher in the durvalumab arm (16.4%) compared with the placebo group (10.6%).39
Head and Neck Squamous Cell Carcinoma (HNSCC)
Multiple phase III trials have evaluated the effects of various combination sequences of ICIs with EBRT on locally advanced (LA) HNSCC. JAVELIN Head & Neck 100 evaluated avelumab in combination with CRT (70 Gy/35 fractions with high-dose cisplatin) in LA-HNSCC compared with CRT alone. Patients in the experimental group were administered a loading dose of avelumab, followed by a concurrent administration with CRT and a maintenance dose. No difference in PFS and OS was noted between CRT alone and CRT in combination with avelumab.40
The IMvoke010 trial evaluated adjuvant atezolizumab vs placebo in patients with LA-HNSCC who underwent multimodal definitive treatment, including surgery or CRT. Interim analysis revealed no improvement in event-free survival and OS with adjuvant atezolizumab.41 Nevertheless, we are still awaiting the results of the ECOG ACRIN EA3161, which is evaluating adjuvant nivolumab after CRT in patients with LA intermediate-risk HPV-positive oropharyngeal carcinoma.42 In the metastatic setting, McBride et al evaluated during a phase II randomized trial the ORR of nivolumab plus SBRT (27 Gy in 3 fractions) compared with SBRT alone. The addition of nivolumab to SBRT did not improve the ORR or led to an abscopal effect.50 For a more comprehensive review of clinical trials investigating the combination of ICIs with EBRT, the readers are referred to existing publication.51
Esophageal Cancers
The phase II/III trial ECOG-ACRIN Cancer Research Group (EA2174) is currently evaluating perioperative nivolumab and ipilimumab in patients with locoregional esophageal and gastroesophageal junction adenocarcinoma. Surgical candidates are administered CRT with or without nivolumab. Following surgical resection, disease-free patients receive nivolumab alone or in combination with ipilimumab.43
KEYNOTE-975 is a phase II trial evaluating the safety and efficacy of pembrolizumab in combination with definitive CRT in patients with unresectable esophageal carcinoma.44 The results from these trials will shed light on the potential role of ICIs in the management of resectable and unresectable esophageal cancers.
Genitourinary Cancers
In prostate cancer, a phase III trial by Kwon et al assessed ipilimumab following palliative radiation of 8 Gy in one fraction to a bone metastasis in patients with metastatic castration-resistant prostate cancer. While the OS benefit was not statistically significant, subgroup analyses highlighted a survival advantage in patients with favorable prognostic factors such as the absence of visceral metastases, normal to slight elevation in alkaline phosphatase and without anemia.45 This study emphasized the importance of patient selection. For muscle-invasive bladder cancer, the phase III trial KEYNOTE-992 is currently ongoing and randomizes patients seeking bladder preservation to concurrent and adjuvant pembrolizumab plus CRT vs placebo plus CRT.46
Cervical Cancer
ENGOT-cx11/GOG-3047/KEYNOTE-A18 is a phase III trial that evaluated concurrent and adjuvant pembrolizumab plus CRT vs placebo plus CRT in patients with high-risk LA cervical cancer. After a median follow-up of 17.9 months, the addition of pembrolizumab to CRT yielded a significant PFS improvement.47
Other Cancers
In a phase II trial, a single fraction of 8 Gy in combination with pembrolizumab showed early response in relapsed multiple myeloma, with 32% of patients experiencing clinical benefit at 3 months. An abscopal response was reported in 20% of all patients, including 3 out of the 7 patients previously treated with CAR T-cell therapy.52 Multiple phase III trials have evaluated the combination of CRT with temozolomide plus nivolumab in glioblastoma with methylated or unmethylated methylguanine-DNA methyltransferase. However, no improvement in survival was observed.53, 54
Limitations and Challenges of Combination Therapy
Combining EBRT with ICIs presents substantial therapeutic potential but also creates significant limitations and challenges. One major hurdle is the immunosuppressive effects of RT. These effects include the activation of regulatory T cells, recruitment of tumor-associated macrophages, and release of immunosuppressive cytokines such as TGF-ß, which collectively reduce the infiltration and activity of cytotoxic T cells within the TME.55 These mechanisms can undermine the clinical efficacy of ICIs. Determining optimal dosing and sequencing strategies is another significant challenge. High radiation doses can potentially be immunosuppressive, while suboptimal doses may fail to induce sufficient tumor cell death or antigen release necessary to prime the immune system.56 The timing of radiation relative to ICIs is also critical. While administering ICIs after radiation can leverage radiation-induced immune activation, the concurrent administration may abrogate the immune system activation and increase the risk of systemic toxicities, including overlapping immune-related adverse events.57 Emerging data also suggest that elective nodal irradiation targeting tumor-draining LNs may interfere with the potential synergism that may ensue from the combination of EBRT with ICIs.58, 59 Thus, lymphatic sparing radiation may be an effective strategy to enhance the synergism between EBRT and ICIs.
Conclusion
The combination of EBRT and immunotherapy has shown considerable potential in improving treatment outcomes across various cancer types. This approach results in enhanced clinical outcomes, including prolonged OS and PFS. However, various challenges persist. Optimizing the radiation dose, field, combination sequence, and timing will be critical for maximizing the potential of EBRT and ICI combinations. Nevertheless, results from current phase III trials are likely to clarify the synergistic relationship between EBRT and ICIs.
References
Citation
Muralidhar A, Idrissou, MB, Sodji QH. Combination of External Beam Radiation Therapy and Immune Checkpoint Inhibitors in Cancer Treatment: Mechanisms, Limitations, and Clinical Applications. Appl Radiat Oncol. 2025;(1):11-25.
doi:10.37549/ARO-D-24-00038
March 1, 2025