Volumetric-modulated arc therapy for malignant pleural mesothelioma after pleurectomy/decortication
Images
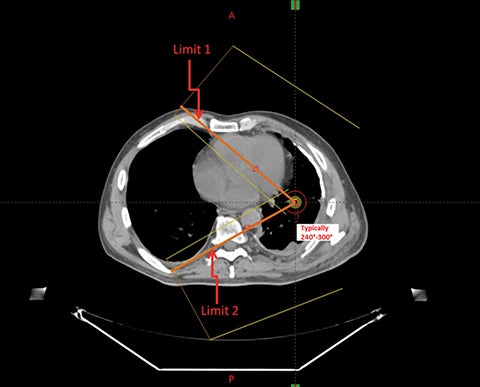
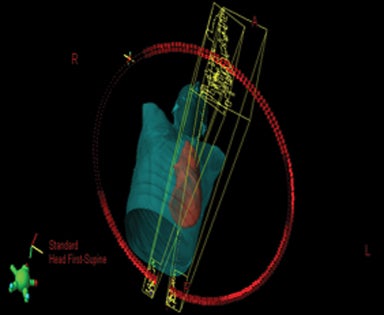
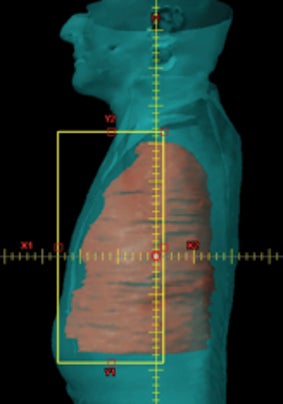
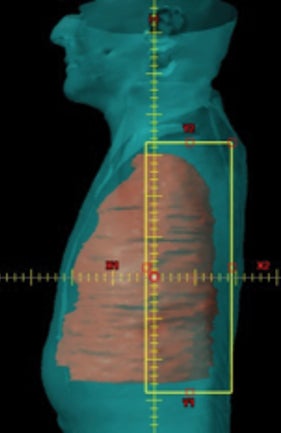
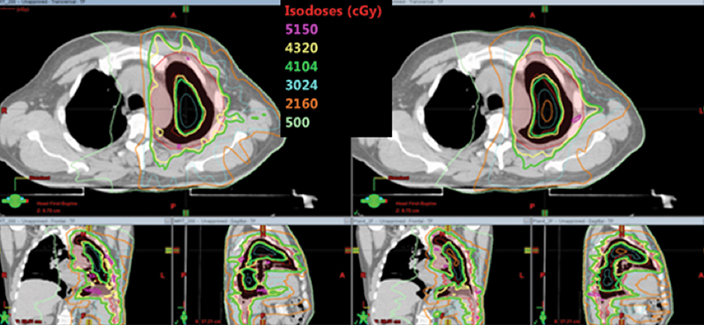
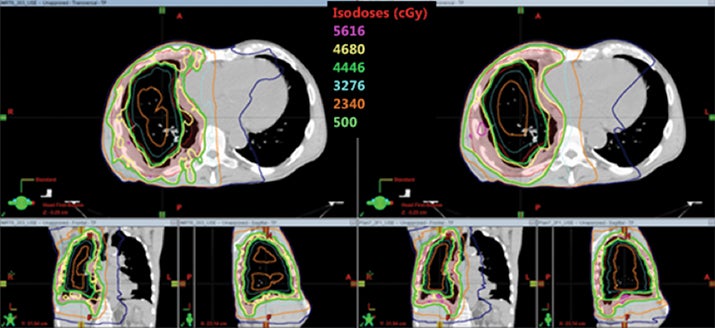
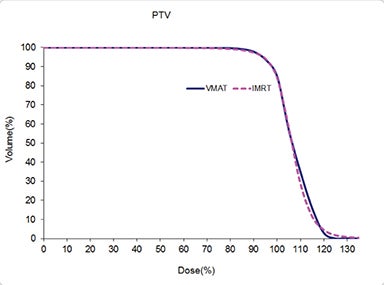
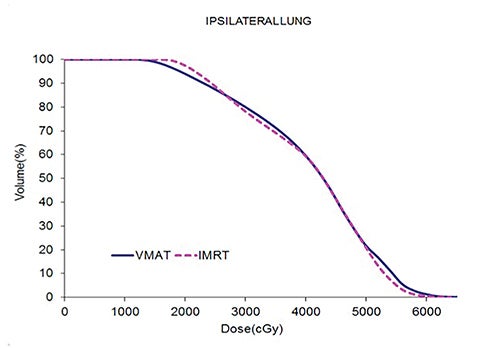
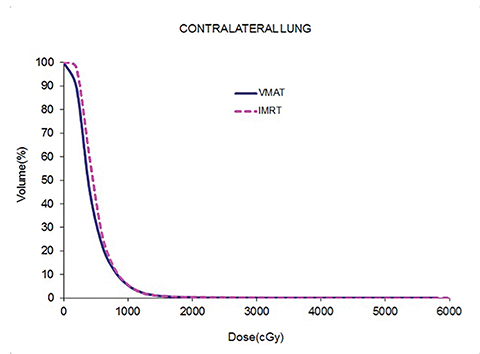
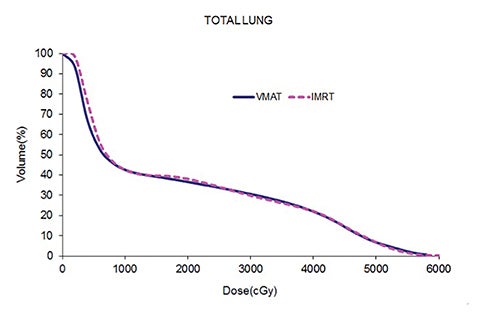
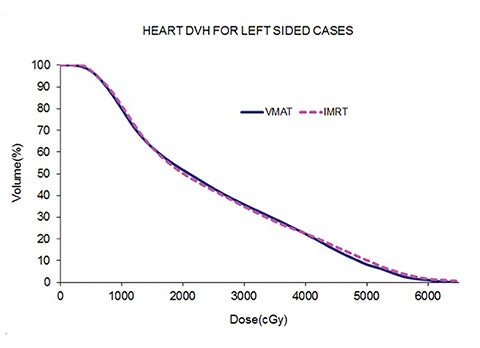
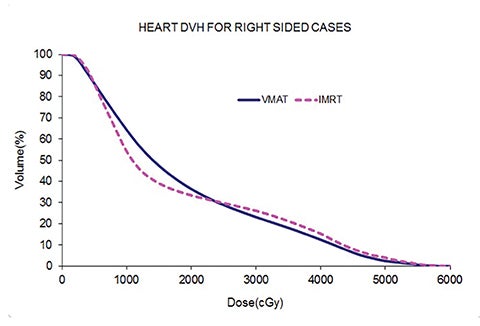
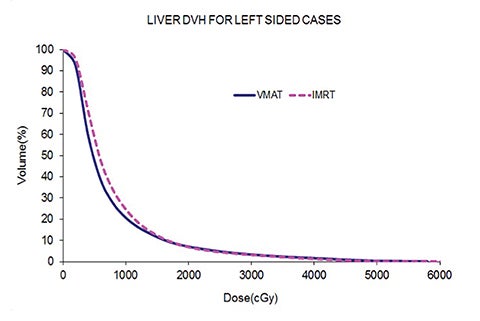
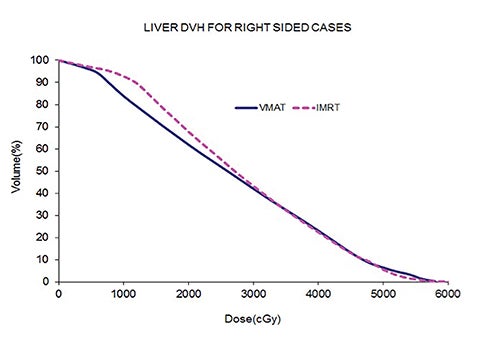
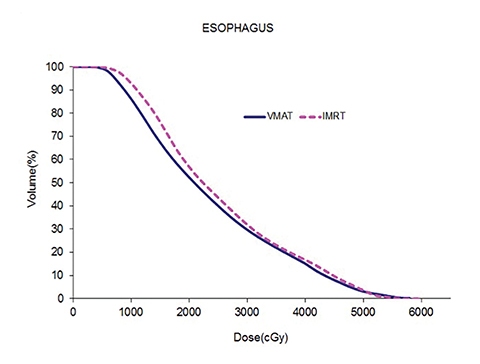
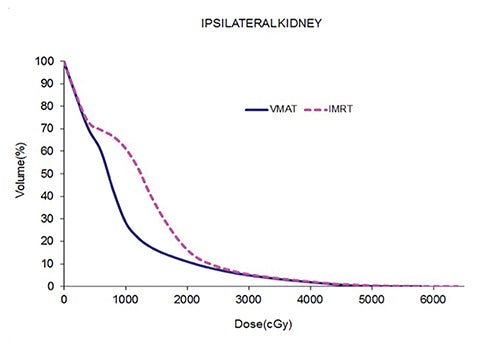
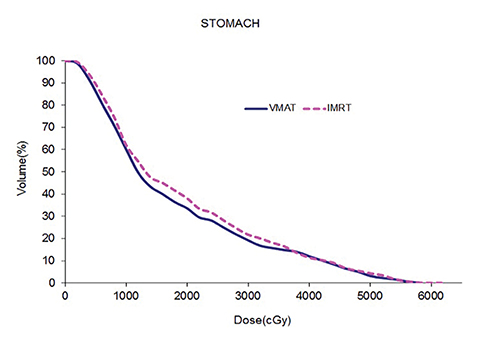
Treatment of malignant pleural mesothelioma (MPM) has historically presented a treatment challenge due to the aggressive nature of the tumor, the size and complexity of the target volume and its proximity to critical organs. Two types of surgery are performed for mesothelioma: extrapleural pneumonectomy (EPP) and pleurectomy/decortication (P/D). EPP involves an en bloc resection of the entire pleura, lung, diaphragm and ipsilateral, half of the pericardium, and is a high-risk surgery for patients with this disease. P/D is a lung-sparing surgery that involves the removal of gross tumor along with the visceral pleura, parietal pleura, pericardium and/or hemidiaphragm if needed for macroscopic complete resection, but spares the underlying ipsilateral lung. Studies have shown that P/D is a less morbid procedure compared to EPP, suggesting a survival benefit and, thus, is becoming increasingly used for these patients.1,2 Surgery alone, however, does not offer adequate long-term local control or survival rates. Multimodality therapy that combines surgery, chemotherapy and radiation therapy has shown favorable clinical outcomes both for patients who have received EPP3 or P/D4 as part of their surgical management. Adjuvant radiation therapy with conventional techniques has been given through anterior and posterior fields that encompass the entire hemithorax.5,6 Since the ipsilateral lung remains in situ after P/D, traditionally these techniques have attempted to spare the lung by adding a block on the central part of the lung.6 Anterior and posterior parts of the chest wall underneath the lung are boosted with an electron field. However, this technique has resulted in disappointing local control, survival rates and high levels of toxicity.5 Since conventional radiation techniques in these patients have been unable to provide adequate local control without compromising toxicity, we must examine advanced radiation planning and delivery techniques. Intensity-modulated radiation therapy (IMRT), a highly conformal radiation delivery technique, has been shown to effectively spare normal tissues while enabling the delivery of higher radiation doses to the tumor, potentially providing safer and less toxic treatments compared to conventional techniques.7 Initial experience with pleural IMRT in 36 patients with 2 intact lungs has shown the safety and feasibility of this technique.8 The risk of grade ≥ 3 pneumonitis was 20%, an acceptable result given the high risk of this patient population. An expanded analysis published using this technique in 67 patients with definitive or adjuvant hemithoracic pleural IMRT showed a median survival of 2 years from the time of diagnosis, with a 1- and 2-year overall survival (OS) rate of 85% and 50%, respectively.9 A recent 2-center phase II trial in which 27 of 45 patients completed hemithoracic IMRT following chemotherapy and surgery reported that 8 of these patients (30%) experienced either grade 2 (n = 6) or grade 3 (n = 2) RP with no grade 4 or 5, and 1 and 2 year OS of 80% and 59% for resectable, and 74% and 25% for unresectable patients.11 Tomotherapy has also been used for pleural IMRT with results showing encouraging survival and acceptable toxicity rates19,20 with reports showing a correlation between the total lung V20 Gy, V30 Gy and mean dose with the incidence of grade ≥ 2 pneumonitis. Of the 69 patients, 6 experienced grade 2 pneumonitis and 7 experienced grade 3 pneumonitis. Radiation therapy delivery using multiple static-field IMRT for MPM is complex and time-consuming.7-11 Moreover, since the treatment fields are large, the planning process often requires splitting the fields, increasing treatment fields twofold, and requiring higher monitor units (MU). Longer treatment times can cause patient discomfort and movement during treatment and, as a result, potential inaccuracies in the delivered dose. Volumetric-modulated arc therapy (VMAT) is a technique that has been investigated and clinically applied for all disease sites including head and neck, thorax, abdomen and pelvis.12 Compared to static-field IMRT, VMAT has been shown to reduce treatment time and MU, making it an attractive RT delivery technique. Dosimetric comparison of IMRT vs. VMAT has been performed for MPM patients with 2 intact lungs,13,14 showing that VMAT produced more homogeneous and conformal dose distributions compared with IMRT, as well as significantly reduced dose to most organs at risk (OARs) without compromising target coverage.
In this article, we assess IMRT vs. VMAT delivery techniques with respect to their dosimetric capabilities, MU and treatment delivery time. We also discuss planning details and the impact of the beam angular arrangement on IMRT planning, as well as arc range and number of arcs on the dosimetric plan quality with VMAT. Using examples of 10 left-sided and 10 right-sided cases, we compare the IMRT vs. the VMAT delivery technique. All plans in this study were designed for delivery on a Varian linac using the Eclipse treatment planning system V 11 (Varian Medical Systems, Palo Alto, California). Statistical testing was done using the Wilcoxon matched-pair sign rank test to compare the significance of dosimetric difference noted between IMRT vs. VMAT. The threshold for statistical significance was p ≤ 0.05.
Patient simulation, volume delineation, dose constraints and planning strategy
Patients were immobilized in a supine position with their arms raised in a customized mold prior to acquiring the CT scan. All patients received a planning CT scan typically at 3 mm slice spacing. A 4-dimensional (4D) CT scan was also acquired at the time of simulation to account for respiratory motion. PET-CT scans were performed at the time of simulation and registered to the treatment planning CT scan to further delineate the target and to include areas of increased FDG (fluorodeoxyglucose) uptake. An initial PTV was defined as a rind, which surrounded the lung/chest wall interface of the entire hemithorax. The superior limit of the PTV was the thoracic inlet at the top of the T1 vertebral body and the inferior limit at the bottom of the L2 vertebral body, including the entire diaphragm and involved lymph node stations. Laterally, the PTV included the parietal pleura along the ribs and medially the mediastinal pleura and the ipsilateral hilum. Visible gross disease based on this CT scan was also included as a part of this PTV with a margin of approximately 8 mm, and the typical width of the PTV rind was 14-16 mm.8 OARs contoured were the ipsilateral lung, contralateral lung, total lung (the union of both the ipsilateral and the contralateral lung), heart, kidneys, liver, stomach, esophagus, bowel and spinal cord. Table 1 summarizes the dose constraints used clinically for the cohorts described in reference 8 (initial experience with pleural IMRT) and 9 (analysis of our patterns of failure and overall survival) and 11 (phase II study on pleural IMRT). Constraints for total lung, contralateral lung and heart are similar to those used during planning non-small cell lung cancer (NSCLC) cases with conventional fractionation, while constraints for the kidney, liver, stomach, esophagus and bowel are similar to those used during planning abdominal tumors with conventional fractionation. Dose constraints were classified as limits or as guidelines. While guidelines could be violated at the discretion of the treating physician, limiting constraints cannot be violated. Our clinical planning strategy was to plan to a prescription dose of 50.4 Gy while meeting target coverage (PTV D95 and V95), hotspots (PTV D05) and OAR constraints with priority given to meeting the limiting dose constraints. Guidelines could be achieved as best as reasonably possible without compromising any of the former criteria. If limiting dose constraints could not be met at 50.4 Gy, the case was discussed with the physician and the prescription dose was reduced by 1 fraction. The case was then re-optimized to see if these constraints were met. This process was repeated until the limiting constraints were met and the prescription dose was determined for the given case.
IMRT
The IMRT planning involves arranging 9 coplanar 6 MV photon beams approximately equispaced over an angular range between 200° to approximately 240°. We define a “lung limit (LL)” range, the angular limits of which are defined by the most medial anterior and posterior edges of the contralateral lung as shown in Figure 1. This range can vary from 240° to 300° depending on the patient’s anatomy. The 200° range includes the ipsilateral hemithorax and extension anteriorly and posteriorly by 10° into the contralateral side. For each case in this study, IMRT plans were performed using 9 static fields uniformly distributed within the 200° range and then within the LL range. A dosimetric comparison of IMRT plans within these 2 ranges was then performed. The isocenter was placed in the middle of the ipsilateral lung. The IMRT plan delivery technique in this study was sliding window.8,9 In our planning experience, we found that we could meet normal tissue dose constraints in Table 1 while maintaining the PTV D95 at no lower than 94%. To form a uniform basis of comparison, all plans were normalized such that 95% of the PTV received at least 94% of the prescription dose. The homogeneity index and conformity index are typically evaluated as D5%– D95%, and as the ratio of the volume of the patient enclosed by 95% of the prescription isodose to the volume of the PTV receiving more than 95% of the prescription dose,15 respectively. Table 2 shows the comparison of IMRT planning with 9 beams arranged in the LL range vs. the 200° range for 20 patients. PTV coverage and hotspots are essentially the same with the 2 types of plans. However, restricting the beam angles to 200° rather than the LL reduced the contralateral lung V5 Gy on average by almost 25%. The dosimetric benefit of restricting the beam angle range to 200° is also seen for other contralateral structures such as the heart for right-sided cases and liver for left-sided cases. Therefore, a more restricted range of beam directions with IMRT is able to adequately cover the target volume while more efficiently sparing normal tissues, especially reducing dose to the contralateral lung. A similar trend has been observed with IMRT for MPM cases that underwent EPP as part of their surgical management.11
VMAT
For VMAT planning, isocenter placement is the same as for IMRT plans and uses 6 MV photon arcs.13 Since the target volumes are relatively large, and due to limitation of the MLC leaf travel within a given treatment field for some treatment machines, typically a minimum of 2 coplanar arcs are required to cover the PTV with asymmetric jaws for these types of VMAT plans.14-16 The jaw settings of the 2 arcs are such that at the maximum dimensional projection of the PTV, both arcs together cover the PTV with a small overlap (around 2 cm) as shown in Figure 2. Since all the IMRT plans used a collimator angle of 0°,8 in this study the collimator angle for each VMAT plan was also set at 0°. Plan normalization methods were kept the same as IMRT. Choice of the arc range in VMAT planning with these 2 coplanar arcs influences the dosimetric plan quality and thereby the ability to meet these dose constraints. Table 3 shows the comparison of VMAT planning using 2 coplanar arcs as described earlier, arranged in the LL range (same as that used in the respective IMRT plan) vs. using the full arc (360°) vs. the 200° range for the same 20 patients. Of the 3 arc ranges, the LL provided the most homogeneous plan for the same PTV coverage. The greatest benefit of the LL range was in sparing the contralateral lung V5 Gy, which was reduced by almost 25% compared to using full arc, and by 55% compared to restricting the arc range to 200°. A benefit of using the LL range was also seen in reducing the mean dose to the total lung. Unlike IMRT, where restricting the beam to 200° better spared the contralateral lung compared to LL, with VMAT, the opposite trend was observed. Restricting the arcs to within a 200° range significantly worsened the dosimetric plan quality with respect to PTV homogeneity and OAR sparing. All other critical organs such as the heart, liver, ipsilateral kidney, stomach, esophagus and bowel were also better spared with the LL arc range when using VMAT. As shown in Table 4, using 4 arcs vs. 2 did not produce a tangible improvement in the dosimetric plan quality and would only increase delivery time.
Comparison of IMRT vs. VMAT
Table 5 compares the dosimetric results with IMRT planning with 9 beams arranged within the 200° range vs. VMAT planning with 2 coplanar arcs within the LL range. Isodose distributions in an axial plane, a sagittal plane and a coronal plane comparing the 2 techniques are shown in Figure 3 for a left-sided case and a right-sided case. Comparison of the dose volume histograms (DVHs) is shown in Figure 4.
Both delivery techniques can produce plans with similar coverage, hotspots, conformity and homogeneity, and are able to meet all the limiting dose constraints of Table 1. The mean total lung dose and V20 Gy are similar among the 2 delivery techniques although are slightly lower with VMAT. Sparing of the contralateral lung with respect to the low dose, ie, V5 Gy, is significantly better with VMAT. Although sparing other critical organs such as the heart, liver, stomach, bowel, cord, esophagus and ipsilateral kidney is comparable between the 2 techniques, VMAT showed a tendency for better sparing. The average delivery time is around 15 minutes for IMRT and 5 minutes for VMAT. VMAT plans were optimized using a maximum dose rate of 600 MU/min. The average MU with IMRT is around 3000, while with VMAT it is almost 1000.
Discussion and Conclusion
The management of MPM continues to present significant challenges. P/D is increasingly becoming the surgical method of choice for patients diagnosed with this disease due to reduced morbidity relative to EPP. The proximity of critical organs including the underlying intact lung can make treatment planning a significant challenge.14 In comparison to IMRT, VMAT is becoming a more popular delivery technique owing to the reduced MU and shorter treatment delivery time, which improves patient comfort, reduces potential error due to patent motion, and improves clinical throughput. Data reported for NSCLC patients has shown that the mean dose to the total lung (MLD) and the total lung V20 Gy are both robust predictors of RP. MLD < 20 Gy and a total lung V20 Gy < 37% are associated with a risk of pneumonitis that is considered acceptable.17,18 Treatment of mesothelioma involves large target volumes, and the incidence of RP is a great concern among these patients. It was recently demonstrated for MPM patients having 2 intact lungs that patients who developed RP had an average MLD ≥ 21 Gy and an average V20 Gy ≥ 40%.19 The corresponding values of these dosimetric parameters in patients who did not develop pneumonitis were 19 Gy and 36%, respectively. Incidence of RP has also been demonstrated in patients who had a contralateral lung V5 Gy ≥ 33%.20 It is therefore essential that dose to the total lung and the contralateral lung be kept as low as possible. Choice of the field/arc arrangement is an influential factor in deciding the plan quality and simultaneously achieving conformal and uniform target coverage and effective OAR sparing. In this article we demonstrate the influence of the choice of angular limits for IMRT and arc range and number of arcs for VMAT for MPM cases that have received P/D. For IMRT, restricting the beams to a 200° range has the highest advantage in sparing the contralateral lung with respect to the low dose, and is recommended over the LL angular range. This observation has also been made in the past with IMRT planning for cases that received EPP as part of their surgical management. Unlike with IMRT, with VMAT, the LL arc range best spares the critical organs and adequately covers the target. Using the 200° arc range produces the least acceptable plans. Moreover, using the full arc range does not improve the dosimetric results over LL range and increases low dose to the contralateral lung, making it an unfavorable choice for planning and delivery with VMAT. With careful choice of angular/arc ranges, both IMRT and VMAT can produce acceptable plans. However, for the same target coverage, VMAT has a tendency to better spare critical organs. Additionally, reduced MU and delivery time makes VMAT a highly attractive treatment option over IMRT, improving both efficiency and patient comfort.
References
- Flores RM, Pass HI, Seshan VE, et al. Extrapleural pneumonectomy versus pleurectomy/decortication in the surgical management of malignant pleural mesothelioma: Results in 663 patients. J Thorac Cardiovasc Surg. 2008;135:620-626.
- Treasure T, Lang-Lazdunski L, Waller D, et al. Extra-pleural pneumonectomy versus no extra-pleural pneumonectomy for patients with malignant pleural mesothelioma: clinical outcomes of the Mesothelioma and Radical Surgery (MARS) randomized feasibility study. Lancet Oncol. 2011;12:763-772.
- Krug LM, Pass HI, Rusch VW, et al. Multicenter phase II trial of neoadjuvant pemetrexed plus cisplatin followed by extrapleural pneumonectomy and radiation for malignant pleural mesothelioma. J Clinic Oncol.2009;27:3007-3013.
- Zauderer MG, Krug LM. Pleurectomy/decortication, chemotherapy and intensity modulated radiation therapy for malignant pleural mesothelioma: rationale for multimodality therapy incorporating lung-sparing surgery. Ann Cardiothorac Surg. 2012;1:487-490.
- Gupta V, Mychalczak B, Krug L, et al. Hemithoracic radiation therapy after pleurectomy/decortication for malignant pleural mesothelioma. Int J Radiat Oncol Biol Phys. 2005;63:1045-1052.
- Rimner A, Rosenzweig KE. Novel radiation therapy approaches in malignant pleural mesothelioma. Ann Cardiothorac Surg. 2012;1(4):457-461.
- Krayenbuehl J, Oertel S, Davis JB, et al. Combined photon and electron three-dimensional conformal versus intensity-modulated radiotherapy with integrated boost for adjuvant treatment of malignant pleural mesothelioma after pleuropneumonectomy. Int J Radiat Oncol Biol Phys. 2007;69: 1593-1599.
- Rosenzweig KE, Zauderer MG, Laser B, et al. Pleural intensity-modulated radiotherapy for malignant pleural mesothelioma. Int J Radiat Oncol Biol Phys. 2012;83:1278-1283.
- Rimner A, Spratt D, Zauderer MG, et al. Failure patterns after hemithoracic pleural intensity modulated radiation therapy for malignant pleural mesothelioma. Int J Radiat Oncol Biol Phys. 2014;90(2):394-401.
- Allen AM, Schofield D, Hacker F, et al. Restricted field IMRT dramatically enhances IMRT planning for mesothelioma. Int J Radiat Oncol Biol Phys. 2007;69:1597-1592.
- Rimner A, Zauderer MG, Gomez DR, et al. Phase II study of hemithoracic intensity modulated pleural radiation therapy (IMPRINT) as part of lungsparing multimodality therapy in patients with malignant pleural mesothelioma. J Clin Oncol. June 20, 2016; DOI:10.1200/JCO.2016.67.2675.
- Teoh M, Clark CH, Wood K, et al. Volumetric modulated arc therapy: a review of current literature and clinical use in practice. Br J Rad. 2011;84:967-1866.
- Dumane V, Rimner A, Yorke, E et al. Comparison of volumetric modulated arc therapy (VMAT) and static intensity modulated radiation therapy (IMRT) for malignant pleural mesothelioma in patients with intact lungs/post pleurectomy. Med Phys. 2012;39:3842.
- Runxiao L, Yankun C, Lan W. A pilot study of volumetric modulated arc therapy for malignant pleural mesothelioma. J Appl Clin Med Phys. 2016; 17:139-44.
- Scorsetti M, Bignardi M, Clivio A, et al. Volumetric modulated arc therapy compared with static gantry intensity-modulated radiotherapy for malignant pleural mesothelioma tumor: a feasibility study. Int J Radiat Oncol Biol Phys. 2010;77:942-949.
- Popescu C, Olivotto I, Beckham W, et al. Volumetric modulated arc therapy improves dosimetry and reduces treatment time compared to conventional intensity-modulated radiotherapy for locoregional radiotherapy of left-sided breast cancer and internal mammary nodes. Int J Radiat Oncol Biol Phys. 2010;76:287-295.
- Graham MV, Purdy JA, Emami B, et al. Clinical dose-volume histogram analysis for pneumonitis after 3D treatment for non-small-cell lung cancer (NSCLC). Int J Radiat Oncol Biol Phys. 1999;45:323-329.
- Kwa SLS, Lebesque JV, Theuws JCM, et al. Radiation pneumonitis as a function of mean dose: an analysis of pooled data of 540 patients. Int J Radiat Oncol Biol Phys. 1998;42:1-9.
- Minatel E, Trovo M, Bearz, A et al. Radical radiation therapy after lung-sparing surgery for malignant pleural mesothelioma: survival, pattern of failure, and prognostic factors. Int J Radiat Oncol Biol Phys. 2015;93:606-613.
- Minatel E, Trovo M, Polesel J, et al. Tomotherapy after pleurectomy/decortication or biopsy for malignant pleural mesothelioma allows the delivery of high dose of radiation in patients with intact lung.J Thorac Oncol. 2012;7:1862-1866.
Citation
Dumane VA, Rimner A, Yorke ED, Rosenzweig KE. Volumetric-modulated arc therapy for malignant pleural mesothelioma after pleurectomy/decortication. Appl Radiat Oncol. 2016;(4):28-37.
December 12, 2016