Stereotactic radiosurgery — The Cleveland Clinic experience and future directions using a Gamma Knife
Images
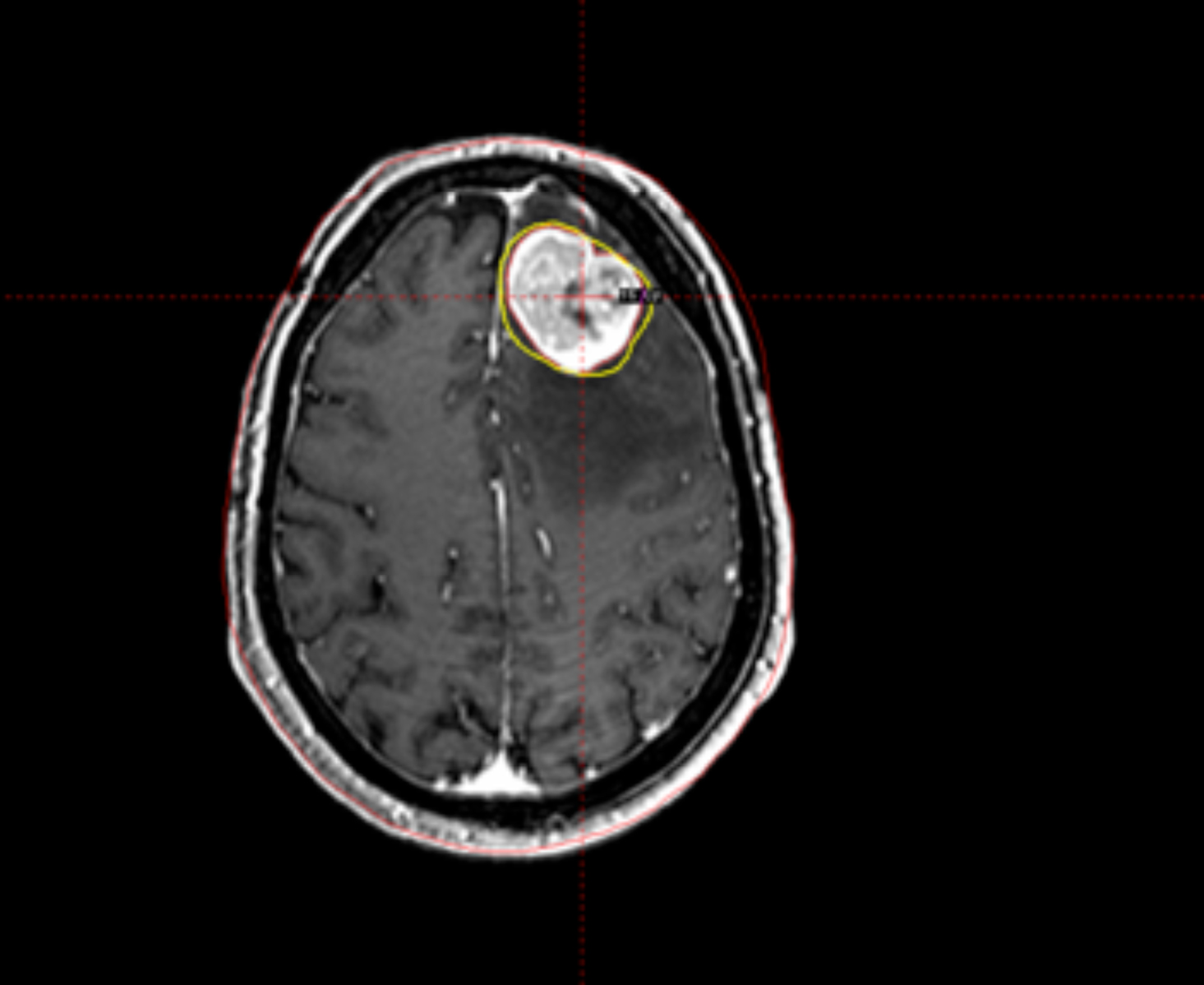
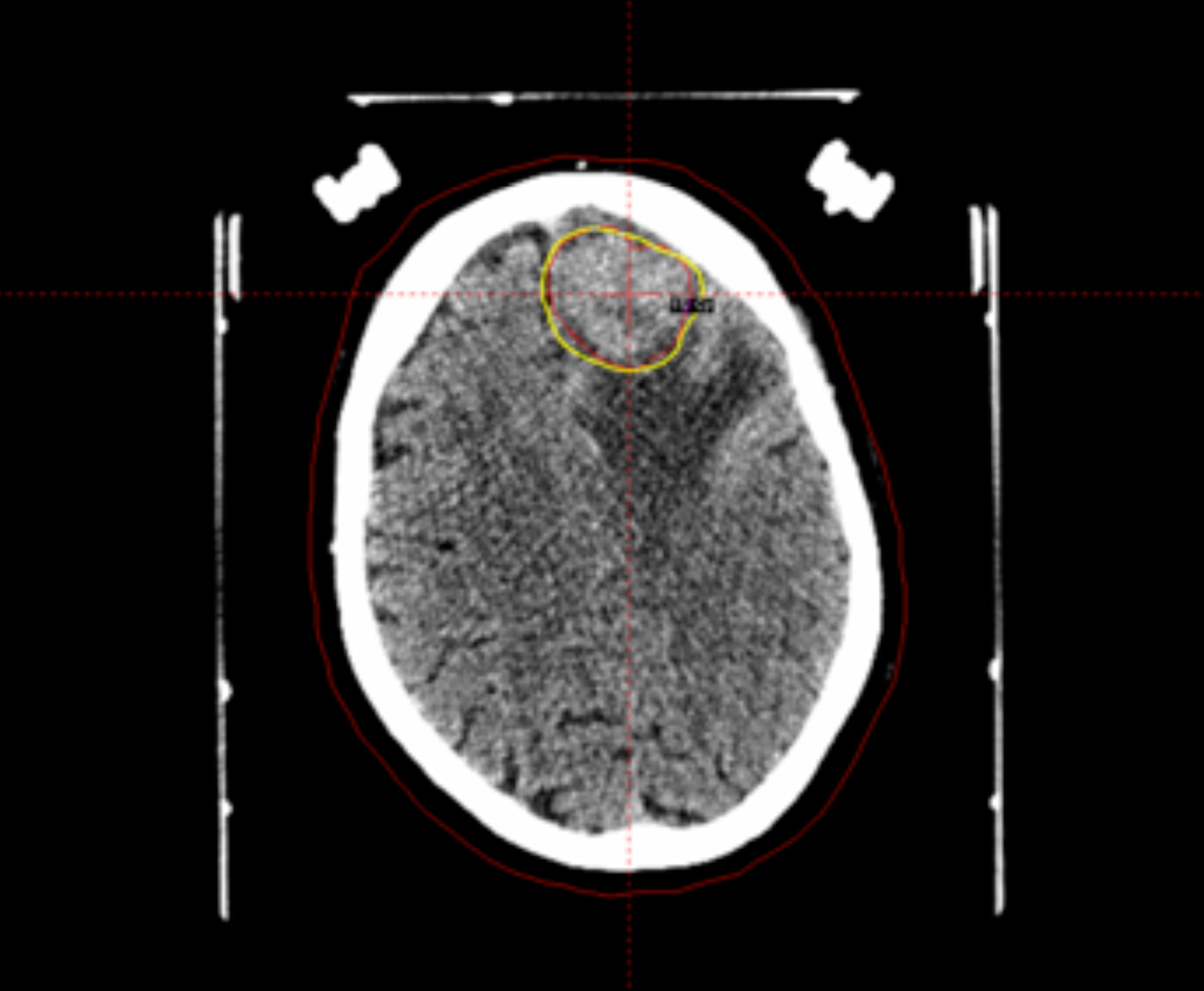
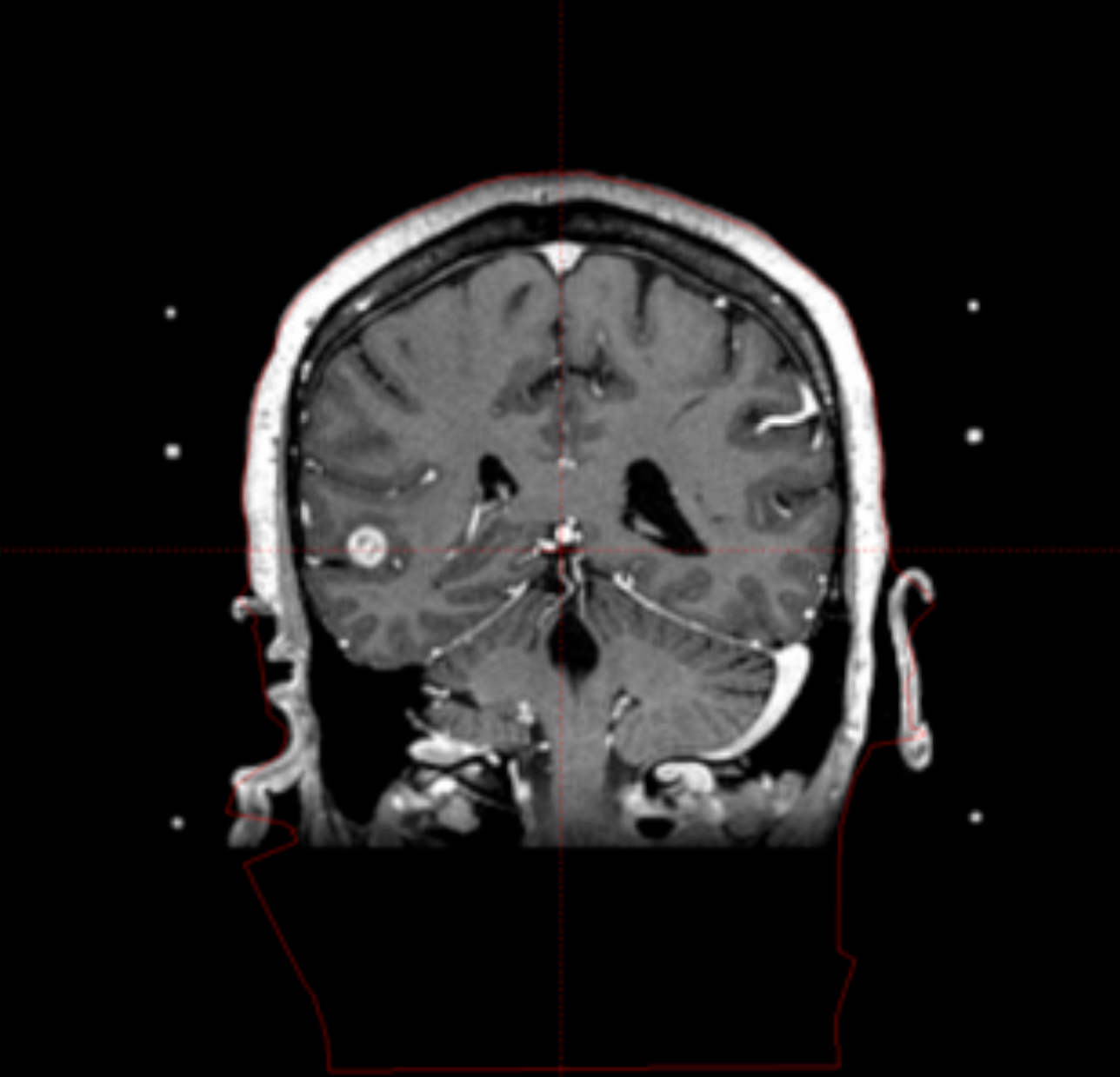
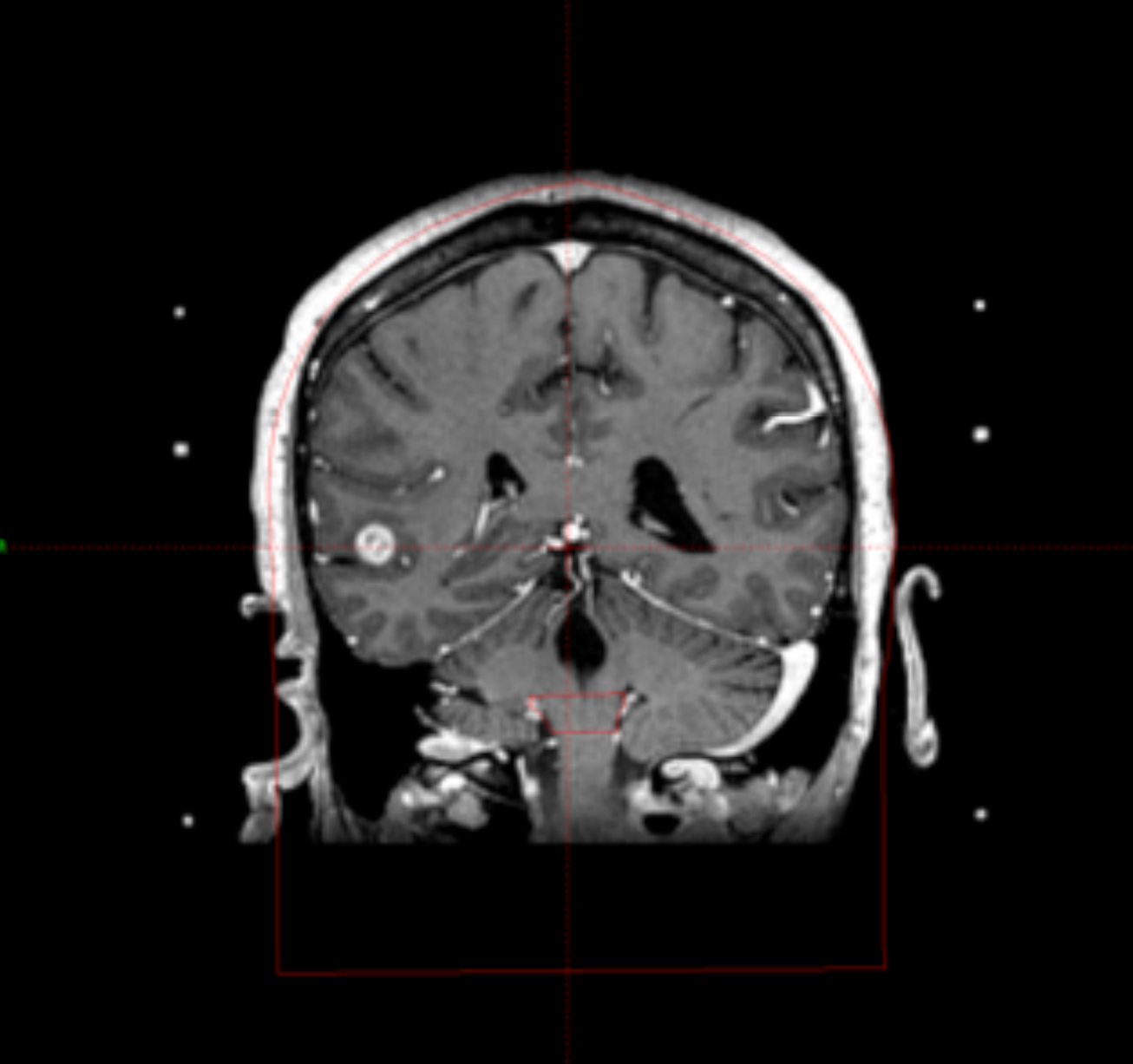
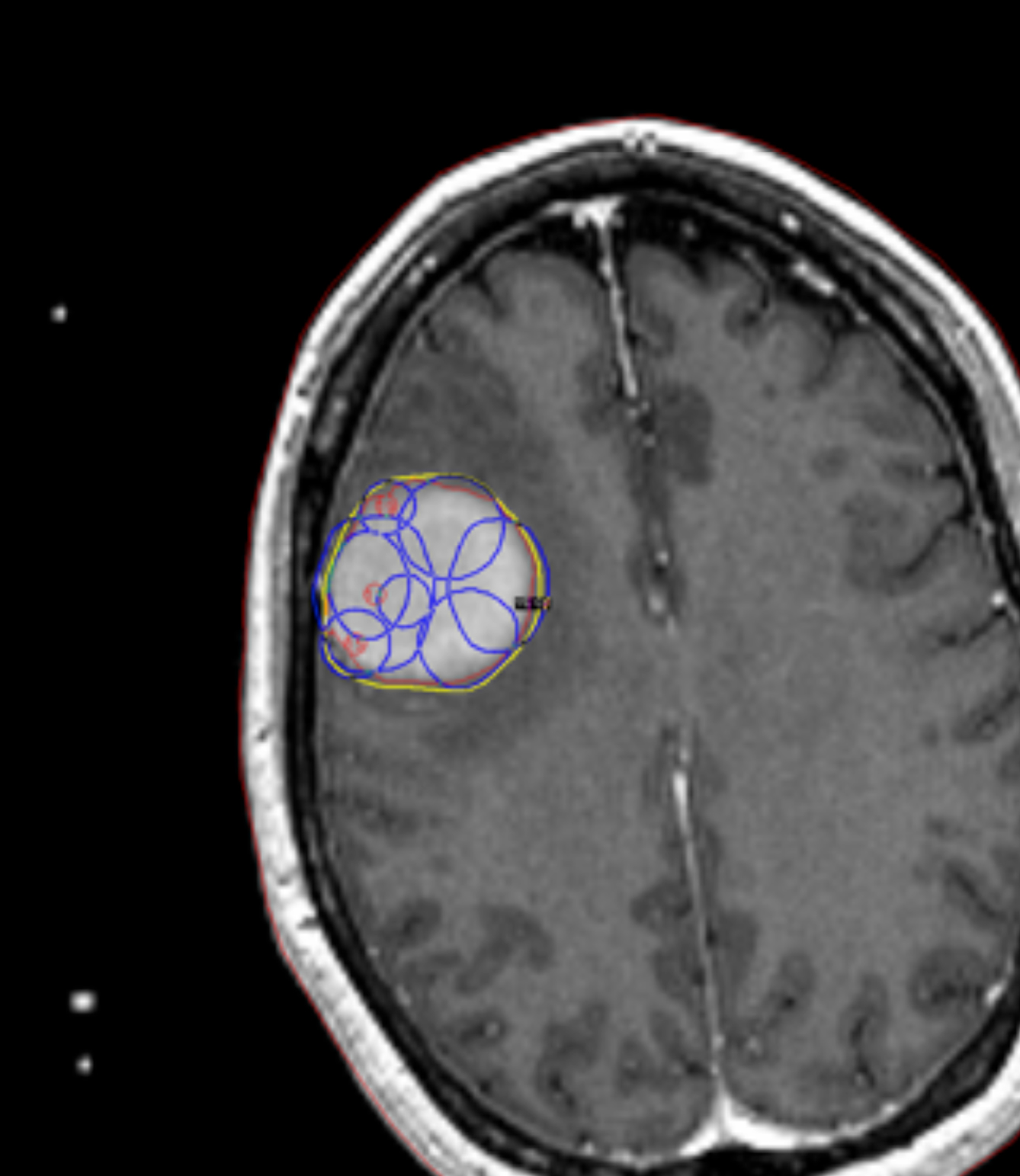
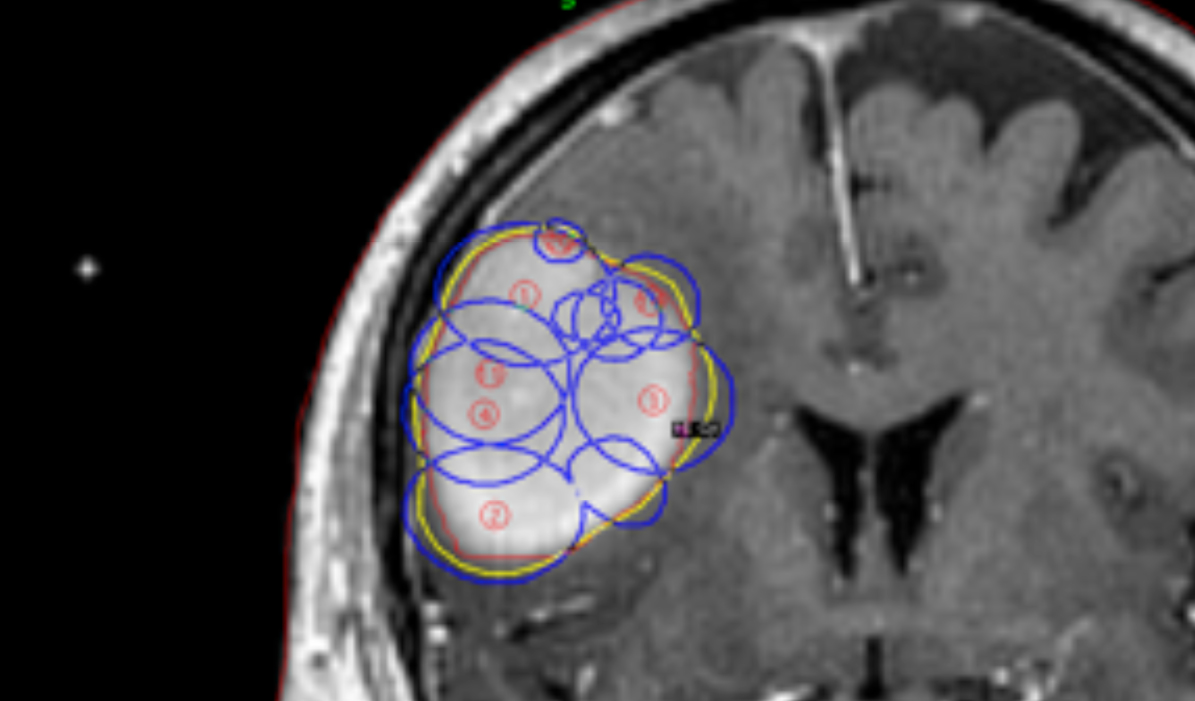
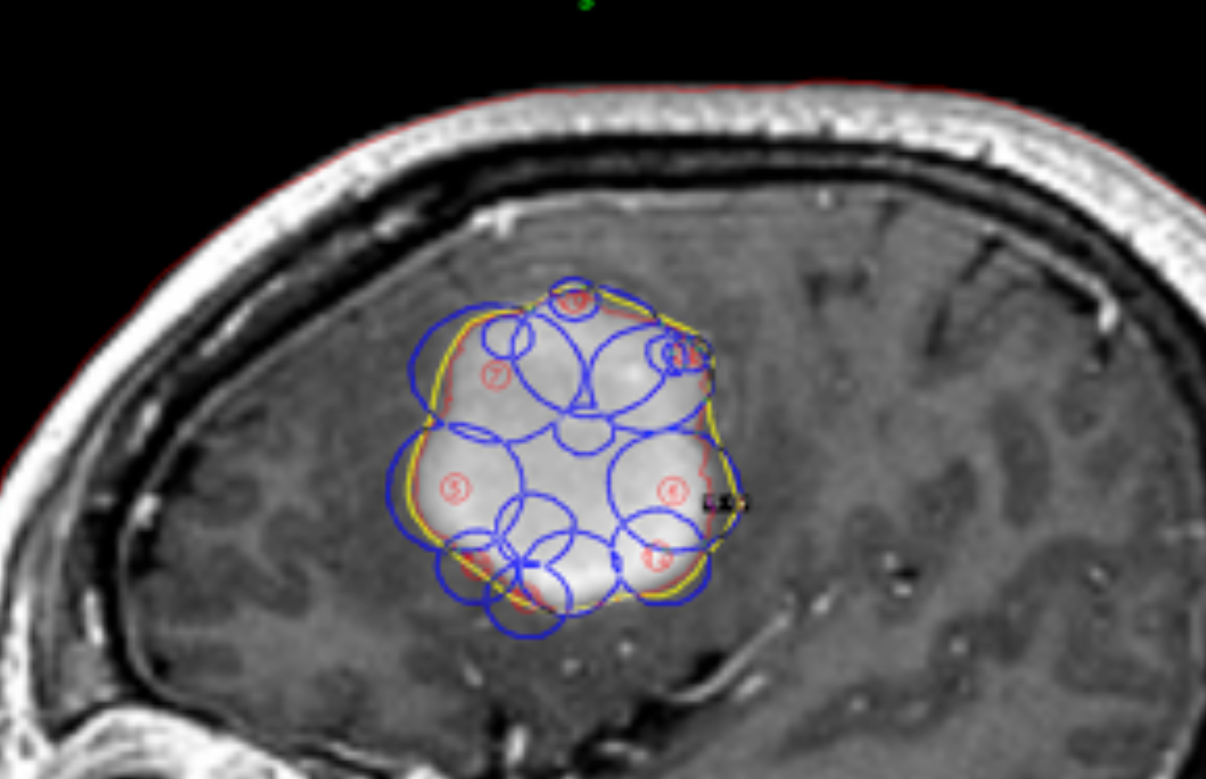
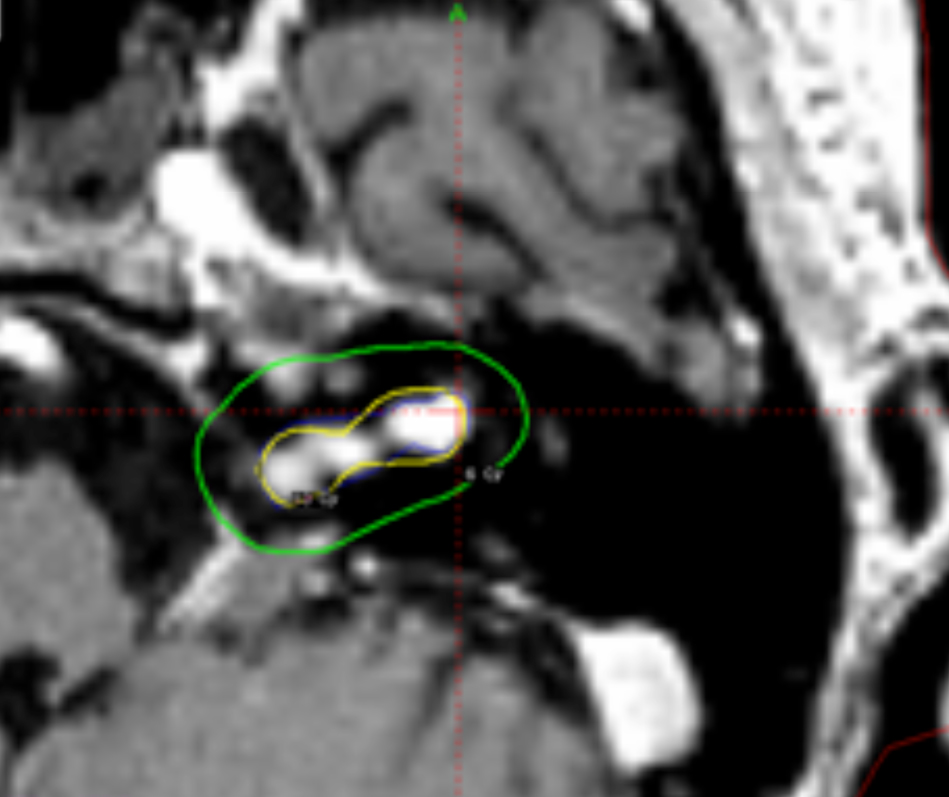
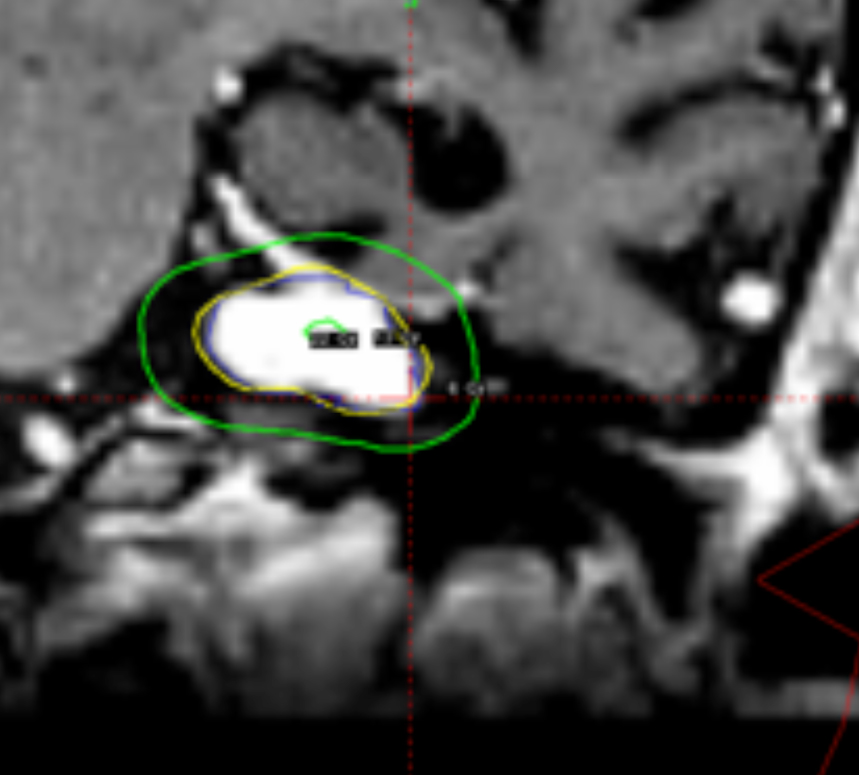
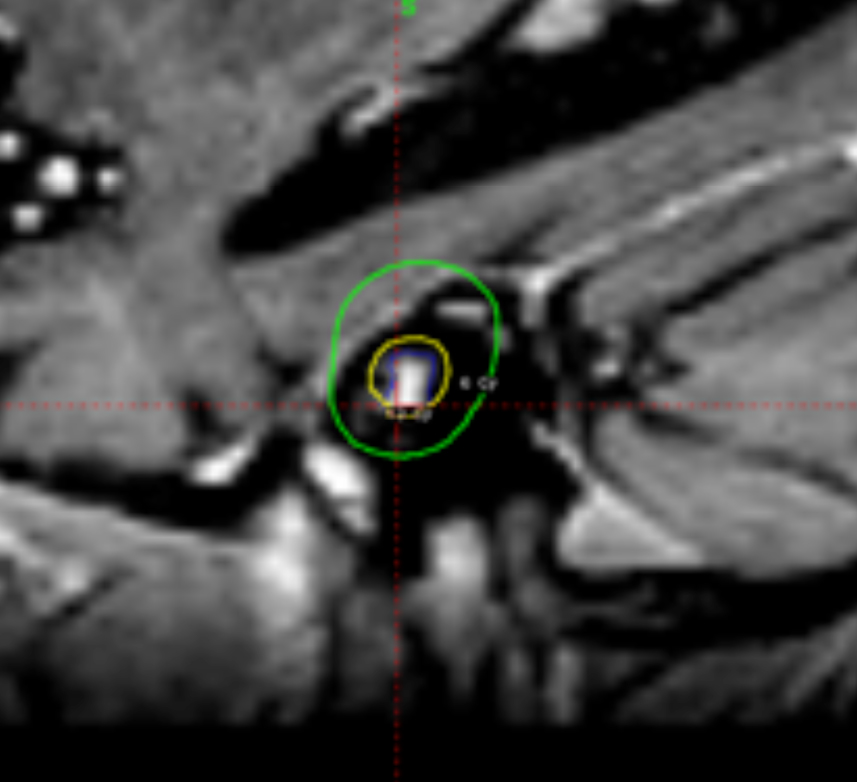
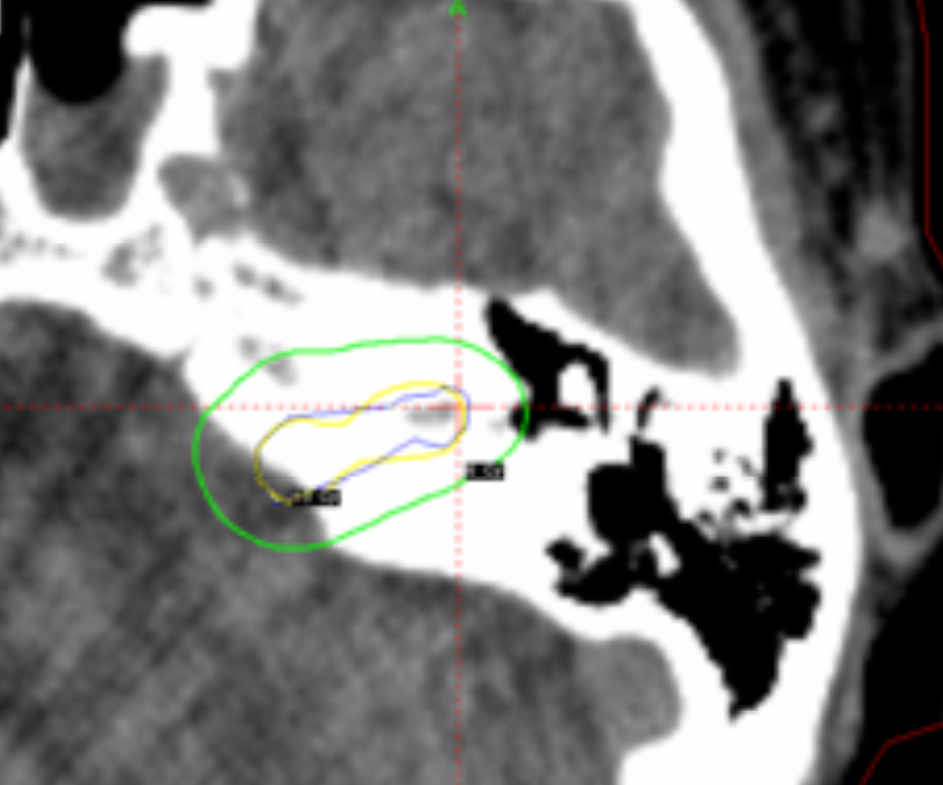
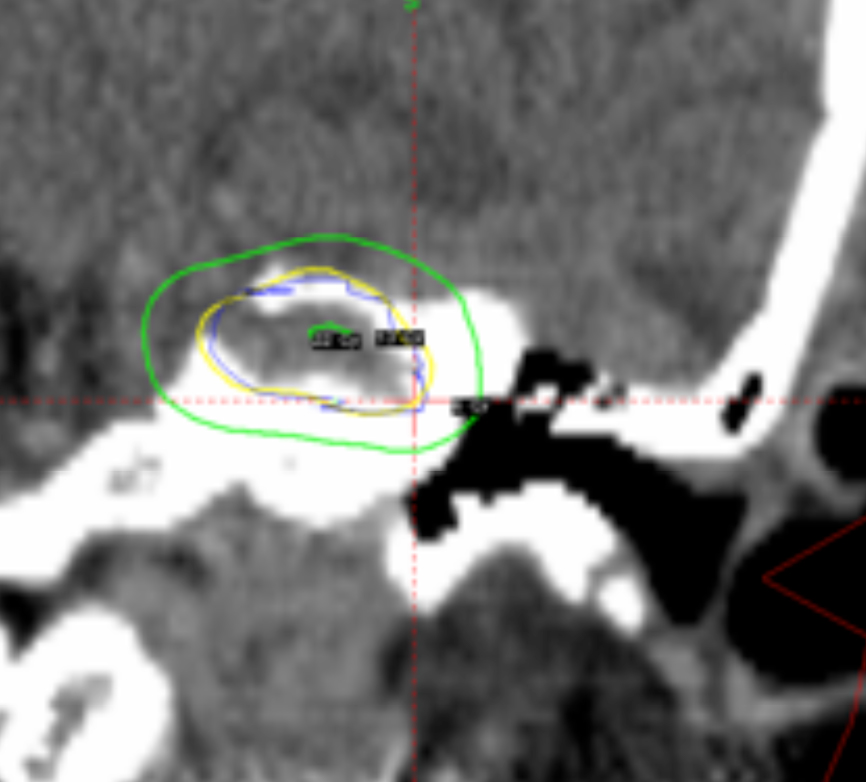
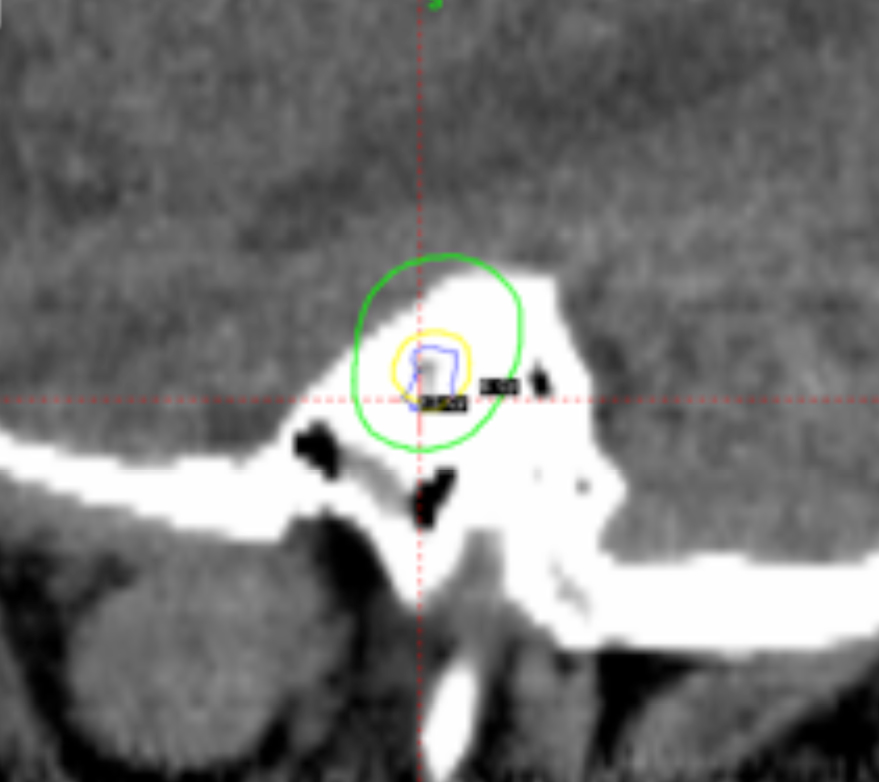
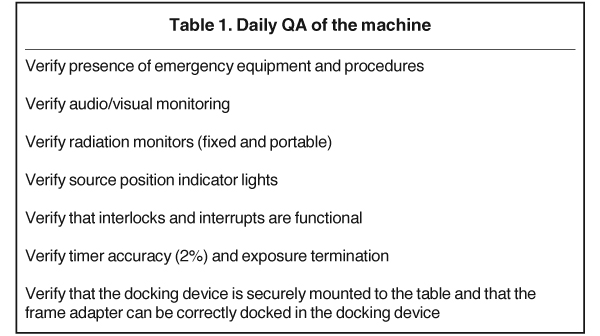
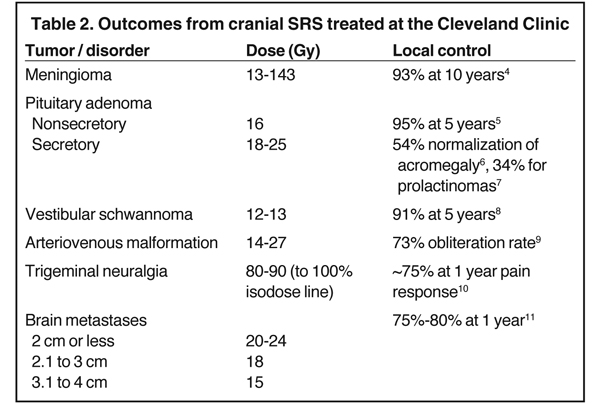
In 1951, Swedish neurosurgeon Dr. Lars Leksell introduced the term radiosurgery, a type of surgery where the surgeon’s knife was substituted with a combination of beams of ionizing radiation.1 In 1967, together with Dr. Larson, a physicist, Dr. Leksell created a fixed-source Cobalt-60 (Co-60) unit, Gamma Unit I, which was the first stereotactic apparatus to perform radiosurgical treatments of intracranial targets.2 Co-60 was chosen as the radiation source because it produces a high-energy beam capable of irradiating deep-seated lesions. Gamma Unit I comprised 170 separate Co-60 beams with 3-mm-x-5-mm slit-shape collimators, and was used to irradiate brain tumors.
Quickly it became obvious that the next generation of Gamma Knife (GK) machines had to produce larger and more spherical radiation fields, which would be a better fit to the typically spherical shape of tumors. In 1975, Gamma Unit II was designed using cylindrical collimators, 4 mm to 20 mm in diameter. The Gamma Knife technology then evolved through several models. In the first model, the 201 Co-60 sources (models A and U) were arranged in a hemispherical array, and irradiation could be delivered using 4 different circular collimator sizes (4, 8, 14, and 18 mm). The drawback of those models was the time-consuming loading and reloading of the Co-60 sources. The unit was redesigned in 1988 (model B) to solve this problem. The process of manually setting the target position was also very labor intensive, so in 1999, models C and 4C, were introduced, which were capable, albeit in a limited range, of shifting the x-y-z target coordinates via an automatic positioning system (APS). However, the APS took up space and reduced the range of motion of the couch, and consequently, limited the access to certain regions of the skull.
To avoid these limitations, the Perfexion model was introduced in 2006; it is based on a single integrated collimator system of 3 different sizes (4, 8, and 16 mm) and an automated couch position. The 192 Co-60 sources of GK Perfexion are split into 8 sectors, potentially better shaping the radiation fields, as these sectors can each move independently with 3 open positions and 1 blocked position. The full automation achieved by the Perfexion GK, including both the positioning of the patient and beam shaping, greatly decreases staff workload and increases patient throughput. The large number of noncoplanar beams, lack of a heavy gantry, short collimator-to-source distance compared to linear accelerators (linacs), and its simplicity are the inherent advantages of the GK stereotactic radiosurgery (SRS) system. It also need to be emphasized that there are some disadvantes using GK compared with the linac based radiosurgery like: the cost of the periodic re-loading of the sources (usually every 5-6 years) and it’s limited use only to the cranial targets. From another side most of the linacs on the market don’t have the same mechanical isocenter accuracy as GK.
GK Perfexion was installed and commissioned at the Cleveland Clinic Gamma Knife Center in July 2007.
Cleveland Clinic Gamma Knife procedure Placement of the Leksell head ring
On the morning of the procedure, the Leksell frame is positioned on the head of the patient, usually using 4 posts/screws under local anesthesia. The Perfexion GK machine allows for central placement of the head frame, fairly independent of the tumor location. Only in 3 cases from more than 2000 patients treated at Cleveland Clinic including patients with multiple targets we were not able to treat all the targets in the same session without re-positioning of the frame. In certain cases of surgical skull defects, a slotted post is used to position the frame with only 3 posts.
MR and CT imaging
One of the most important aspects of radiosurgery is accurate imaging of the target. Both high-resolution magnetic resonance imaging (MRI) and computer tomography (CT) are typically used for GK treatment planning at Cleveland Clinic. The use of 2 independent imaging modalities confirms positioning and helps minimize errors from spatial distortions of the MRI. Additionally, angiograms are used for arteriovenous malformations (AVM) and vascular tumor radiosurgery. The dedicated radiation treatment software for Gamma Knife (Gamma Plan) allows the coregistration of preframe MRI with postframe CT with the CT fiducial device attached using the mutual information volume algorithm. Gamma Plan version 10 allows for restriction of the co-registration to the area close to the region of interest instead of the whole head. This can greatly improve the accuracy and ease of the co-registration process (Figure 1). The advantages of the co-registration technique include:
- no distortions from the Leksell frame on MRI scans,
- easier for patient to tolerate the MR scan without a frame on his head, and
- decreased workload for Gamma Knife personnel on the day of treatment.
>
Neurosurgeons perform the co-registration at Cleveland Clinic. The Gamma Plan doesn’t quantify for the user how accurate the coregistration is, so the neurosurgeons have to rely on a good visual match in the vicinity of the target. With this approach the CT scanners have to go through a rigorous QA program to check for the quality of its images.
In our center, for MRI of most lesions, a 3-dimensional (3D) volume gadolinium-enhanced acquisition is used with T1 FLAIR sequence at 256 x 256 matrix, 1-mm slice thickness and no gap. CT imaging is mostly done with contrast at 512 x 512 matrix and 1 mm to 2 mm slice thickness. Angiograms, when necessary, are corrected using digital subtraction techniques.
Measurements of the skull geometry
Traditionally, the patient’s skull geometry is defined by measuring the distances from the center of the frame to 24 preselected points on the skull using the skull scaling instrument. Those measurements are susceptible to human error and sometimes are not very accurate in simulating the skull, especially when the patient’s skull is deformed because of the bone resection. In our center, an existing patient CT scan allows us to use a relatively new feature of the Gamma Plan—extracting the contour of the patient skull from the CT image (Figure 2).
Target localization
The MR and CT are visually compared to see if spatial distortions more than 1 mm are present in the MR images. This procedure is usually performed by a physicist with the participation of the neurosurgeon.
Target delineation
Target delineation is accomplished by a neurosurgeon. In certain cases, the targets are drawn on both MR and CT studies and compared.
Treatment planning
This is a combined effort by each member of the team consisting of the neurosurgeon, the radiation oncologist, and the physicist, to produce the best plan. The plans are compared using dose volume histograms (DVH) for the outlined targets and organs at risk (OAR). The plan is designed with the prescription to the target at 50% isodose line or higher. In certain cases the new inverse treatment planning feature of Gamma Plan version 10 is used, which can ease the job of planning, especially for the coverage of big and complicated targets (Figure 3).
From the DVH, a number of plan indices are calculated and compared between different plans:
- Conformity Index (CI) = PIV/TV, where PIV is the prescription isodose volume and TV is the target volume.
- Gradient Index (GI) = PIV0.5/PIV, where PIV0.5 is the volume getting half the prescription dose.
- Homogeneity Index (HI) = Dmax/Dmin, where Dmax and Dmin are maximum and minimum dose respectively.
The goal for the planning is to achieve 100% target coverage, or very close to that (no less than 98%). In the process of planning, the plan with the smaller CI and less dose to the critical organs is chosen. When the plan is finalized, the physicist checks the plan for target coverage, dosimetric indices, and dose distribution. Another important check is the deliverability of the treatment as planned, without risks of collisions with the machine. The physicist also makes sure the dose rate used for the calculations in the Gamma Plan is accurate by comparing it to tabulated values of decayed dose rate from initial calibration of the sources and also by running a quick calculation. Typical daily quality assurance (QA) checks on the Gamma Knife machine, which are done before treatment of the first patient of the treatment day, are presented in Table 1.
Treatment implementation
GK Perfexion usually creates just one run per patient treatment even for multiple targets. Separate runs are necessary when different gamma (neck) angles are used. Before actual treatment the radiation oncologist is checking that the frame is stable on the patient by pushing the frame up and down.
Clinical uses
The Gamma Knife can be used to treat malignant and benign tumors, vascular disorders, and functional disorders. Malignant tumors include brain metastases and recurrent malignant gliomas. Benign tumors include vestibular schwannomas, meningiomas, pituitary adenomas, and glomus tumors. Vascular disorders are typically AVMs. Functional disorders include trigeminal neuralgia and Parkinson’s disease. At Cleveland Clinic, the decision to treat is made by the neurosurgeon and radiation oncologist, and which is facilitated through the Tumor Board that meets twice a week. Table 2 is a summary of outcomes of the most common indications treated at the Cleveland Clinic.
Future Developments
Collapsed-cone convolution algorithm for the gamma plan
Elekta has already implemented the collapsed-cone convolution (CCC) algorithm for Gamma Plan, which requires a whole-head CT scan. The collapsed cone convolution algorithm has some advantages compared with the traditional tissue-maximum ratios algorithm (TMR) that assumes the head uniformly as water, and can achieve more accurate calculations of the dose distribution in the field of GK radiosurgery. The main advantages of CCC are: a) it more accurately models build-up effects; b) it takes head heterogeneity into account; and c) it increases tissue correction and accuracy to dose calculation for targets near air cavities and bone.
Despite those advantages, the CCC algorithm is rarely used in clinical practice because most institutions use only MRI studies for planning without CT scans. Also, the CT protocol has to be adjusted because of the particular requirements for field of view (FOV). Using our database, it was shown that the TMR algorithm systematically overestimates the actual physical dose delivered compared with recalculations of the same plans using CCC algorithm by about 6% to 8 %.12 An example of a 2% loss of dose coverage for an acoustic schwannoma tumor, recalculated by CCC algorithm after being calculated originally by TMR algorithm, is shown in Figure 4.
A relocatable frame system for multiple-session GKRS
Gamma Knife surgery traditionally was limited to a single-session treatment with the rigid frame-based technique. It is known that certain brain tumors may have a better outcome using fractionated radiation treatments. This is typically how linear accelerator-based systems treat.13 Elekta has released the Extend System that allows the GK Perfexion System to treat multisession GK procedures. This is a noninvasive frame that uses a vacuum-assisted bite block device positioned on a carbon-fiber frame. The reposition check tool, which consists of a Plexiglas frame and electronic measurements probes, is used to position the patient’s head in the same place for each subsequent treatment. The mean intrafractional positional difference is about 0.5 mm with a standard deviation of 0.3 mm using the Extend System, when it was studied in 10 patients with 36 fractional treatments.14
Cone-beam computed image guidance system for GKRS
Another recent development in GK radiosurgery was described by Ruschin et al.15 The authors integrated a custom-built cone-beam computed tomography (CBCT) installation for image guidance in GK Perfexion System. This can improve the precision of fractionated radiation treatment delivery. A kV cone beam CT system was integrated with a GK machine and consists of the 40-cm x 30-cm flat-panel x-ray detector and an x-ray tube placed on an imaging arm. The images of the phantom showed that the maximum targeting error was 0.4 mm. The system needs additional clinical evaluation before it can be fully implemented in clinical practice.
Conclusion
The Cleveland Clinic Gamma Knife procedure and clinical results are summarized in this article. The continuing developments in this field can greatly expand the possibilities of Gamma Knife radiosurgery and solve some of its previous limitations.
References
- Leksell L. The stereotactic method and radiosurgery of the brain. Acta Chir Scand. 1951;102:316-319.
- Leksell L. Stereotaxis and radiosurgery: An operative system. Thomas Publishers, Springfield, IL, 1971.
- Balagamwala EH, Suh JH, Barnett GH, et al. The importance of the conformality, heterogeneity, and gradient indices in evaluating Gamma Knife radiosurgery treatment plans for intracranial meningiomas. Int J Radiat Oncol Biol Phys. 2012;83:1406-1413.
- Flickinger JC, Kondziolka D, Maitz AH, Lunsford LD. Gamma knife radiosurgery of imaging-diagnosed intracranial meningioma. Int J Radiat Oncol Biol Phys. 2003;56:801-806.
- Sheehan JP, Starke RM, Mathieu D, et al. Gamma Knife radiosurgery for the management of nonfunctioning pituitary adenomas: A multicenter study. J Neurosurg. 2013.
- Jagannathan J, Yen CP, Pouratian N, Laws ER, Sheehan JP. Stereotactic radiosurgery for pituitary adenomas: A comprehensive review of indications, techniques and long-term results using the Gamma Knife. J Neurooncol. 2009; 92:345-356.
- Sheplan Olsen LJ, Robles Irizarry L, Chao ST, et al. Radiotherapy for prolactin-secreting pituitary tumors. Pituitary. 2012;15:135-145.
- Murphy ES, Barnett GH, Vogelbaum MA, et al. Long-term outcomes of Gamma Knife radiosurgery in patients with vestibular schwannomas. J Neurosurg. 2011;114:432-440.
- Pollock BE, Gorman DA, Coffey RJ. Patient outcomes after arteriovenous malformation radiosurgical management: Results based on a 5- to 14-year follow-up study. Neurosurgery. 2003;52:1291-1296;discussion 6-7.
- Kanner AA, Neyman G, Suh JH, Weinhous MS, Lee SY, Barnett GH. Gamma knife radiosurgery for trigeminal neuralgia: Comparing the use of a 4-mm versus concentric 4- and 8-mm collimators. Stereotact Funct Neurosurg. 2004;82:49-57.
- Chao ST, Barnett GH, Vogelbaum MA, et al. Salvage stereotactic radiosurgery effectively treats recurrences from whole-brain radiation therapy. Cancer. 2008; 113:2198-204.
- . Neyman G, Somell B, Nordstrom H. Comparison of collapsed cone convolution with the traditional tissue maximum ratio algorithm for dose calculation in a Gamma Knife radiation treatment planning system. Medical Physics. 2013;40, 6:337 (AAPM annual conference proceedings, 2013, Indianapolis).
- Tuniz F, Soltys S, Choi C, et al. Multisession Cyberknife steroatactic radiaosurgery of large, benign cranial base tumors: Preliminary study, Nerosurgery. 2009;65:898-907.
- Schlesiner D, Xu Z, Taylor F, et al. Interfraction and intrafraction performance of the Gamma Knife Extend system for patient positioning and immobilization. J Neurosurgery (Suppl). 2012;117:217-224.
- Ruschin M, Komljenovic P, Ansell S, et al. Cone beam computed tomography image guidance system for a dedicated intracranial radiosurgery treatment unit. Int J Radiat Oncol Biol Phys. 2013;85:243-250.