Real-time Prostate Gland Motion and Deformation During CyberKnife Stereotactic Body Radiation Therapy
Images
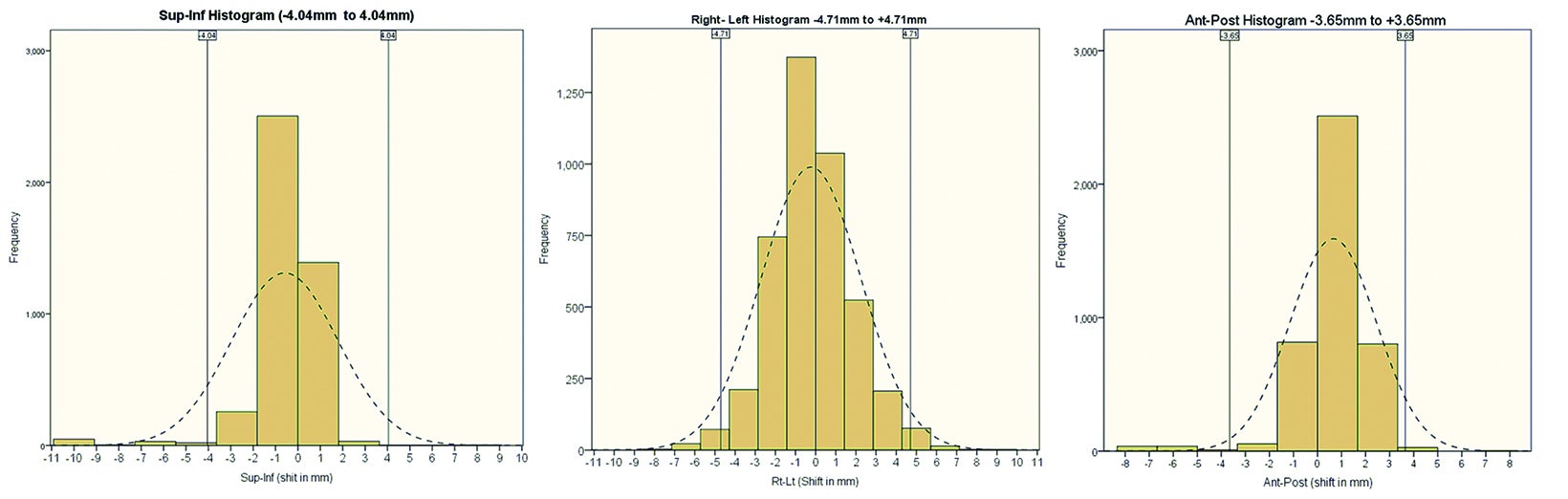
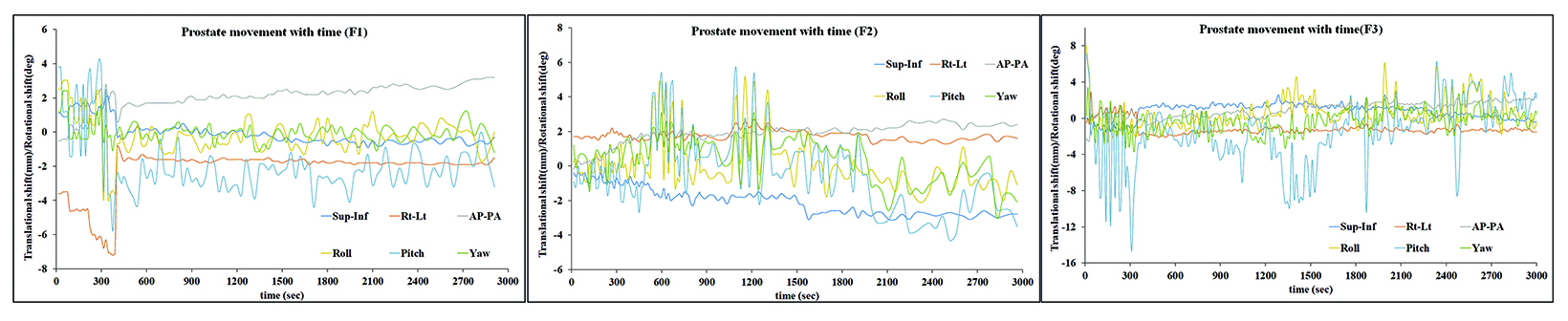
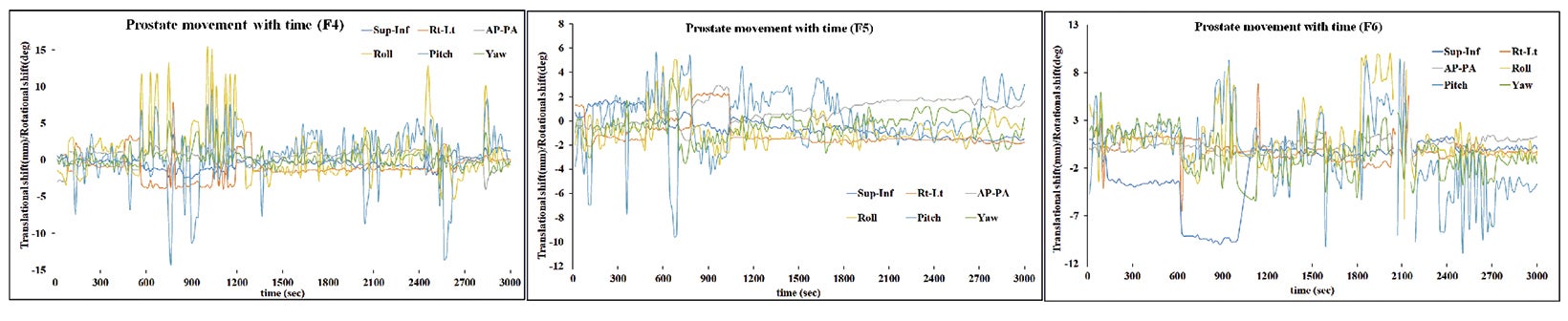

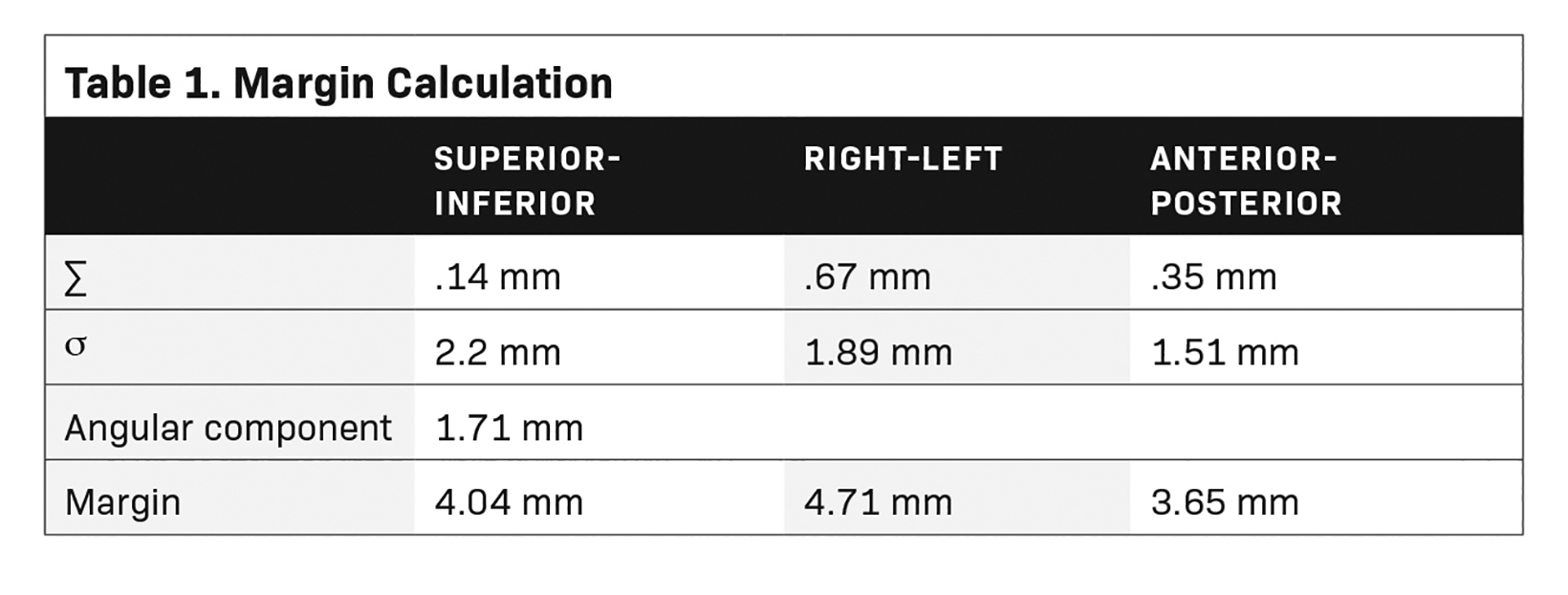
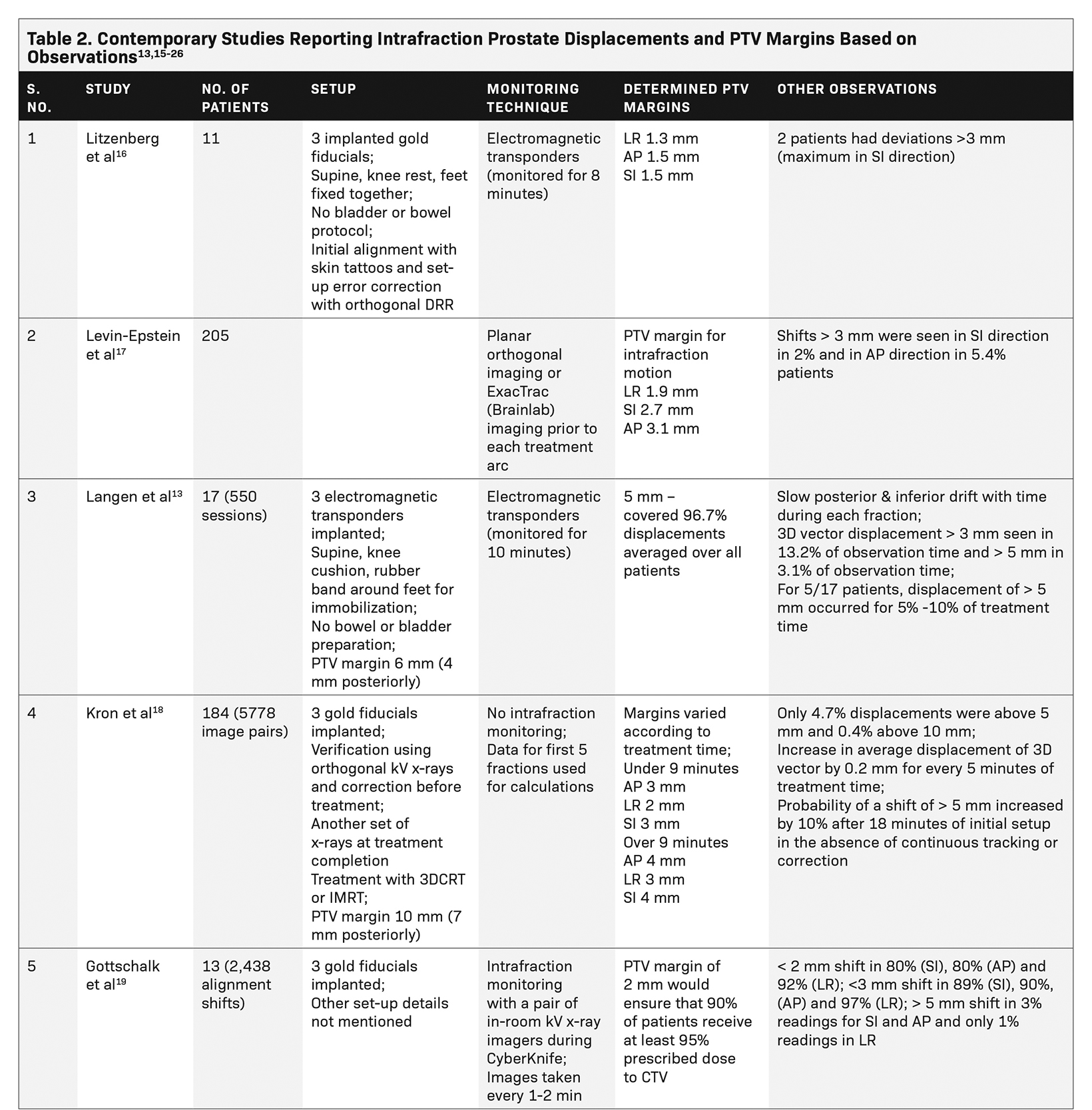
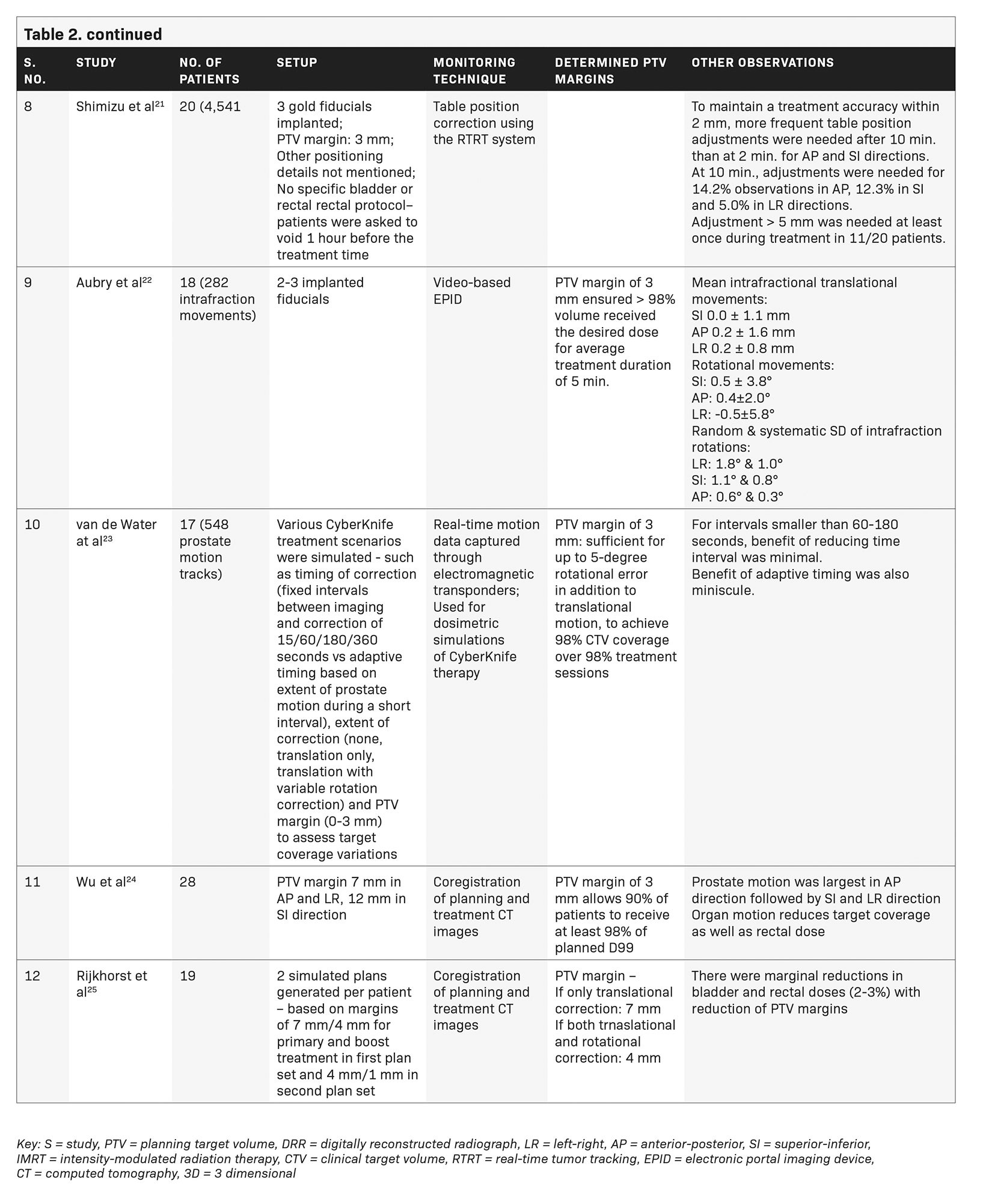
Abstract
Purpose: To analyze intrafraction prostate motion during radical CyberKnife stereotactic body radiation therapy (SBRT) and calculate the planning target volume margins needed to ameliorate errors related to this intrafraction motion.
Materials and Methods: We analyzed the data for 10 consecutive patients with prostate cancer treated with the Accuray CyberKnife (54 fractions, 5,465 alignment shifts in target position). Real-time alignment shifts during delivery of SBRT fractions were obtained by in-room image tracking of 3 to 5 (minimum 3 required for tracking) gold seed fiducials implanted in the prostate. The intrafraction target motion was analyzed, and the margins required to compensate for the real-time motion were calculated using margin recipe formulae.
Results: The translational mean ± standard deviation (SD) in left-right (LR), anterior-posterior (AP), and superior-inferior (SI) directions was 1.65 ± 2.17 mm, 1.24 ± 1.55 mm and 1.13 ± 2.1 mm, respectively. The rotational mean ± SD was 1.2 ± 1.33, 2.17 ± 2.14, and 0.99 ± 0.87 degrees in roll, pitch, and yaw, respectively. Motion in LR, AP, and SI directions was less than 2.0 mm in 78.1%, 86.73%, and 92.21% of readings, respectively, and less than 3.0 mm in 85.9%, 96.5%, and 95.7% of readings, respectively. A margin of 5 mm in LR, 4 mm in both SI and AP directions would ensure that ≥ 95% of patients receive at least 95% of the prescribed dose.
Conclusions: Our dataset constitutes among the largest series of intrafraction prostatic motion during SBRT. The observed intrafraction prostate motion is highest in the LR and SI directions, and least in the AP direction.
Keywords: CyberKnife, intrafraction motion, prostate, stereotactic body radiation therapy
Radiation therapy (RT) dose escalation in curative treatment of prostate cancer has yielded better disease control, but with a corresponding higher probability of organ-at-risk (OAR) complications.1-3 Image guidance before each treatment fraction using various in-room imaging technologies (electronic portal imaging device [EPID], cone-beam computed tomography [CBCT], etc.) may help reduce the interfraction set-up errors. However, the prostate gland undergoes significant physiological spatial motion and volumetric deformation during the course of a radiation fraction. Both these interfraction and intrafraction variations are accounted for by adding a planning target volume (PTV) margin during RT planning. Hypofractionated treatments or dose escalation studies involve a relatively higher fractional dose and need a much sharper peripheral dose fall-off compared with conventional fractionation and delivery techniques, thus necessitating a reduction in PTV margins to minimize normal tissue adverse effects.4,5 This requirement is addressed by various techniques that measure variations in daily setup and internal organ motion during a treatment fraction, either by assessing organ motion directly or through surrogates such as implanted fiducials (ultrasound, EPID, CBCT, electromagnetic transponders in Calypso [Varian]).6-13 Strict and efficient image guidance may help limit PTV margins considerably, and thus enable the dual goals of dose escalation and normal tissue sparing. Although techniques such as volumetric-modulated arc therapy (VMAT) and the current hot-topic FLASH radiation therapy have potential to reduce these uncertainties by lowering the fraction duration, we will not be discussing this aspect as the present study deals only with a specific SBRT system and related real-time intrafraction motion not adequately explored in the aforementioned strategies.
At our institution, we use the CyberKnife system (Accuray Inc.) for stereotactic body radiation therapy (SBRT). This system uses frequent real-time stereoscopic x-ray imaging of implanted fiducials during treatment delivery to monitor changes in prostate position. Each pair of stereoscopic images acquired is used to compute the center of mass (CM) of implanted fiducials, and this forms a surrogate for intrafraction prostatic movement. This study is an analysis of individual patient data of 10 consecutive patients treated with CyberKnife for definitive prostate cancer radiation and seeks to quantify intrafraction prostate motion. The purpose of presenting this data is to guide PTV margins based on observed translational and rotation motion. We also calculated the deformation of the prostate gland during the treatment.
Materials and Methods
All 10 patients (54 fractions) received SBRT with the CyberKnife VSI System using gold-seed fiducial-based tracking between December 2018 and December 2019. SBRT was used as monotherapy or in combination therapy as a prostate boost with standard pelvic radiation (using conventional fractionation) on a linear accelerator (linac). Following informed consent, 3 to 5 (minimum 3 required for tracking) gold fiducials were placed in the periphery of the prostate gland (1 at the prostate apex, 2 near the base on the right and left side, 1 to 2 fiducials in the transverse plane midway between the apex and base on the right and left sides) under transrectal ultrasound guidance per CyberKnife protocol (> 2 cm and > 15 degrees apart, within 6 cm of each other). Usually, at least 3 of these fiducials would be usable for tracking the prostate during SBRT. After waiting to 5 to 7 days to allow for any possible fiducial movement/migration, noncontrast RT planning CT scans (slice thickness 1 mm, no superposition of slices, 512 × 512 pixel matrix, pitch 1) with the patient immobilized in the supine position with a customized full- body vacuum cushion (empty bladder, optimal rectal emptying) were obtained and co-registered with thin section volumetric MR images (T2-weighted and T1 contrast sequences) acquired in the supine position for target delineation. This step was undertaken first regardless of whether an SBRT boost preceded or followed the linac-based RT. Adequate bowel preparation was ensured prior to simulation and before delivery of each fraction to minimize errors in prostate localization and to prevent interference with daily imaging during treatment.
Specifically, the patients were counseled for a low-residue diet during the first consultation and advised to have adequate hydration throughout the day. Those with pre-existing constipation were additionally advised to take laxatives and a charcoal-simethicone combination to allow a regular bowel movement and minimize bowel gas, starting 3 to 4 days before simulation and continuing until the last fraction. Occasionally,
a phosphate enema was given if the simulation topographic scan showed the rectum loaded with feces, in which case simulation was re- attempted after an hour of the enema. The prostate clinical target volume (CTV) was delineated on the primary CT dataset (co-registered with axial T2- and T1-contrast sequence volumetric MRI with 1-mm slice thickness) with the help of a radiologist specializing in prostate imaging. An isotropic 3-mm margin around the CTV was given to generate a final PTV, which was prescribed to receive a dose of 1,650 to 1,800 Gy in 3 fractions for an SBRT boost (4 patients, 3 fractions per patient), or 3,500 to 4,200 cGy in 7 fractions when delivering radical SBRT to the prostate (6 patients, 7 fractions per patient).
The 2 Gy equivalent dose (EQD2) for the SBRT plan was calculated using the formula, EQD2 = n × d × (α/β + d)/(α/β + 2), keeping in mind the limitations of dose equivalence in high fractional doses. MultiPlan software (Accuray Inc.) was used for treatment planning whereby inverse planning helped achieve the prescribed target dose while respecting normal tissue constraints for the given dose fractionation. Plans were then evaluated for adequate target coverage, dose heterogeneity and conformality index. Strict patient-specific quality assurance was ensured for accurate and smooth treatment delivery.
The CyberKnife SBRT system employs a robotic, arm-mounted, miniaturized 6 MV linac with 6 degrees of freedom. Translations from 0.6 to 10.0 mm, and rotations in roll (2.0 degrees), pitch (5.0 degrees) and yaw (3.0 degrees), in either direction, can be corrected by the robotic arm without needing to move the patient couch. The planning system generates multiple pairs of digitally reconstructed radiographs (DRRs) after plan approval, which are used as references for treatment verification. The treatment room has 2 in-room, ceiling-mounted kV x-ray sources placed at 45 degrees to the vertical along with 2 flat-panel detectors on the floor. These aid real-time imaging of the implanted fiducials during treatment delivery, thus allowing detection and subsequent correction of intrafraction shifts. In tempo adaptive imaging system of CyberKnife (time-based adaptive image guidance) is used for prostate SBRT to assist in the frequency of tracking (minimum interval between 2 consecutive image acquisitions from 5 to 150 seconds) and thereby corrects nonpredictable intrafraction target motion. In tempo imaging helps determine the best frequency of imaging depending on the instability in the prostate position. Every projection pair acquired by the cameras is co-registered in 6-dimensional space with a reference DRR image pair using a complex tracking algorithm, and intrafraction target motion data are generated.
Once the patient is set up in the treatment position using in-room lasers, verification images are acquired and, within seconds, co-registered with the planning DRRs to detect set-up errors and correct them by couch movement before initiating treatment. During treatment, real-time set-up errors are continuously recorded, and appropriate adjustments are performed by the robotic arm, without needing to move the patient. However, larger deviations lead to greater uncertainties in the accuracy of correction by the robot; hence, it is advisable that these deviations are kept to a minimum during treatment. At our institution, we use a threshold of 5.0 mm for translational shifts. If the calculated shift exceeds this threshold, the robot automatically pauses the treatment, and remote-assisted couch movement is performed to correct gross movements, so the robot can continue fine adjustments once the shift is within the threshold limit.
To analyze our data on prostate motion, we retrieved the treatment log files (containing data on fiducial tracking with time) from the CyberKnife treatment localizer system for 10 patients, accounting for a total of 5,465 intrafraction shift datasets; these were reviewed for this study. By using in-house MATLAB version R2015a (MathWorks) programming, the translational, rotational and deformation shifts of the fiducials were obtained with respect to the fiducial position on the RT planning CT scan. Statistical analysis was done using IBM SPSS software (version 24). Histograms in descriptive statistics were obtained with Gaussian distribution trendline for translational and rotational shifts. A scatter line graph was plotted between fiducial position and time through the treatment duration for the entire dataset of 1 patient with 7 fractions.
Margin Calculation
Standard deviation (SD) of the mean for the individual shifts was calculated for systematic uncertainty (Σ). For random errors (σ), root mean square of the individual patient’s SD was used. The traditional Van Herk margin recipe was modified to include the margin for the angular component.
For angular margin, the following formula was used:
Angular margin (mm) = xsin(Θ) + ycos(Δ) + (z/xy) × tan(Γ)
Θ - roll; Δ - pitch; Γ - yaw (all in degrees)
Total Margin = 2.5Σ + 0.7σ + angular component
We took the initial 5 minutes of each fraction for systematic error, and the rest of the variation during treatment for random error calculations.14 Mean value of the angular linear component was added to individual translational margin to account for the rotational movement of the fiducials.
The CyberKnife system records the rigid body error (RBE) data for every fiducial used for tracking at every time stamp. The RBE at a particular time is defined as “the distance of a fiducial from its corresponding position on planning CT after the system has assessed the best translational and rotational transformation through a rigid registration of the acquired in-room projection images with CT- generated DRRs.”15
Results
The translational mean (± SD) for intrafraction motion of the prostate in superior-inferior (SI), anterior-posterior (AP) and left-right (LR) directions was 1.24 mm (± 1.55 mm), 1.65 mm (± 2.17 mm), and 1.13 mm (± 2.10 mm), respectively. The rotational mean (± SD) for roll, pitch, and yaw was 1.20 (± 1.33), 2.17 (± 2.14) and 0.99 (± 0.87) degrees, respectively. Intrafraction prostate motion for > 97% readings was ± 4.01 mm (SI), ± 3.65 mm (AP) and ± 4.71 mm (LR). Figure 1 shows the histograms depicting the range of intrafraction translational movement of the COM of the fiducials in all 3 dimensions (1A SI, 1B LR, 1C AP).
We observed intrafraction prostate motion under 2 mm in 92.21% (SI), 86.73% (AP), and 78.10% (LR) readings, and less than 3 mm in 95.7% (SI), 96.50% (AP) and 85.90% (LR) readings, respectively. We observed intrafraction prostate motion of over 5 mm only in 1.8% readings, and this was only in the SI direction.
It is important to note that in our patients, treatment delivery began only after the computed shift was lower than the preset 5 mm threshold for fiducial tracking. Throughout treatment, automatic adjustments to incident beam were made by the robot to compensate for deviation in target localization. If the deviation exceeded 5 mm, then treatment stopped automatically, and the patient realigned such that translational error returned within the threshold limit before restarting treatment. Hence, the observed values do not represent the mean target motion magnitude during the entire treatment fraction. However, it is also important to note that the frequency of such erratic larger magnitude shifts is significantly less compared with the majority of the motion recorded during the treatment time of a single fraction. This practice may be responsible for < 2% incidence of > 5 mm translational shift in our study. These shifts account for the actual prostate motion during most of the treatment duration, barring a few erratic large movements.
Based on the above data, the calculated margins for translational motion were 4.04 mm (SI), and 3.65 mm (AP), and 4.71 mm (LR), respectively. On the other hand, rotational prostate movement as noted from fiducials is random; it is observed during the initial part of treatment in most patients and midtreatment in others. The detailed contribution to the margin from systematic and random errors and the angular component (deformation) are depicted in Table 1. Figure 2 represents the prostate movement for a representative patient’s treatment fractions over time (F1 through F7 represent fractions 1-7 for a patient). From our analysis, we surmised that time interval between 2 consecutive intrafraction image acquisitions should preferably be less than 20 seconds for the first 380 seconds of treatment and not exceed 35 seconds throughout the treatment.
RBE calculated for the fiducial shifts was 0.59 mm (± 0.29 mm) for fiducials 1-2, 0.51 mm (± 0.29 mm) for fiducials 1-3, and 0.73 mm (± 0.38 mm) for fiducials 2-3. Figure 3 shows the rigid body error for our patients in A) SI, B) LR, and C) AP directions. Average treatment time for all patients per fraction was 48.29 +/- 5.31 min (range, 45 to 56 min).
Discussion
Most protocols for prostate SBRT use PTV margins of 5 mm circumferentially (and 3 mm posteriorly toward the rectum). Assuming that real-time determination of intrafraction movement of the target during CyberKnife may help reduce PTV margins and make dose escalation safer, we used a 3 mm circumferential margin for our patients based on the study by Litzenberg et al who demonstrated a reduction in PTV margin to less than 2 mm if intrabeam adjustments or continuous tracking were performed.16 However, we observed in our study that although a 3 mm PTV margin may cover more than 95% of intrafraction motion in SI and AP directions, it served less than 90% motion in the LR direction. We determined that a margin of 4 mm in SI and AP directions and 5 mm in the LR direction (after correction of set-up errors at the outset) would ensure that 95% of the planned dose would be delivered to at least 95% patients. It is possible that other factors such as positioning and immobilization techniques, bowel and bladder protocol variations, or patient weight and general fitness may contribute to differences in target motion between various intrafraction motion monitoring techniques.
Table 2 lists several studies that assessed intrafraction prostate displacements and reported on PTV margins based on their observations.13,15-26 A large patient database of a VMAT study using 4 half arcs with an average treatment duration of 15.6 minutes showed that a 3-mm margin is desirable for intrafraction translational motion correction. Rotational motion was not studied, and in this study, the relative displacements decreased over subsequent arcs after the first correction.17
Langen et al tracked the real-time prostate motion during intensity-modulated radiation therapy (IMRT). They noted a slow posterior and inferior drift in prostate position with time during a fraction, possibly due to muscle relaxation or change in rectal contents. Sudden and transient anterior and superior movements were seen, possibly attributable to peristalsis. Lateral movements were infrequent. Large interpatient variations were noted. Frequency of displacements grew with increasing time from start of treatment, with only 2% of the observations with displacement exceeding 3 mm during the first minute, increasing to 23% during the tenth minute. They recommended that the gap between initial setup and treatment initiation should be minimized as far as possible.13 Kron et al made similar observations on the rise in intrafraction motion according to increasing treatment duration.18 We did not notice a similar drift, possibly due to couch adjustments whenever the motion was out of range (preset 5 mm limit) but this is an important observation suggesting that a shorter treatment time would minimize additional errors.
The CyberKnife study by Gottschalk et al reported that a PTV margin of 2 mm would give at least 95% target coverage for more than 90% patients.19 Another study of CyberKnife SBRT by Xie et al also used a preset threshold of 5 mm for couch correction, as in our study. They reported intrafraction motion similar to our results with average shift vector length of 2.61 ± 1.94 mm.15 However, they studied only translations and deformations; rotational shifts were not considered. Additionally, their dataset was much smaller compared with our dataset. Similar to the findings of Langen et al,13 they noted increasing motion with time since treatment initiation. Motion > 2 mm increased with increasing time since initiation of therapy, reiterating the need to start treatment quickly after setup. Their RBE value was within 1.5 mm, suggesting a relatively smaller contribution from deformation. Our data also notes the RBE value of the fiducials to be well within 1.5 mm. Yu et al, based on their experience with Synchrony (Accuray Inc.) tracking, have suggested respiratory motion tracking of implanted fiducials to mitigate errors due to respiratory motion, although none of the other CyberKnife studies including ours have used respiratory tracking for the prostate.20
Shimizu et al classified patients as those with large motion (> 5 mm displacement within 10 minutes), increasing (> 5 mm displacement noted after 10 minutes) or steady (no displacement > 5 mm) based on magnitude and timing of observed intrafraction movement during real-time tumor tracking (RTRT), and recommended that monitoring duration may be tailored to type of motion.21
Aubry et al used video-based EPID to determine intrafraction prostate motion during IMRT (average treatment time 5 minutes).22 Rotational data were recorded but not corrected. Our study noted relatively larger rotations than their study in coronal (LR), sagittal (SI) and transverse (AP) planes at 1.20 (± 1.33), 2.17 (± 2.14) and 0.99 (± 0.87) degrees, respectively. Rotation exceeding 3 degrees in the Y axis was found in 10.9% readings, suggesting a significant rotational component of intrafraction motion in our dataset.
van de Water et al, in their CyberKnife simulation study, determined a 60- to 180-second imaging interval as optimum for maintaining CTV coverage, with little contribution of adaptive imaging.23 In contrast, a much shorter imaging interval of 35 seconds throughout treatment and in tempo imaging were found useful for motion management in our patients. Simulations of in tempo imaging were possibly not able to fully determine the true impact of variations that occur during an actual treatment session. Our study also assessed the impact of deformation and its correction, though RBE was < 1 mm for our patients.
Several studies have tried to assess the impact of intrafraction motion on organ-at-risk doses although we have not been able to address that with the CyberKnife system.24-26 Wu et al studied intrafraction translational motion and sagittal rotation during 3DCRT, and determined that intrafraction motion compromised PTV coverage and reduced rectal dose.24 Rijkhorst et al, in their simulation study, also showed the increasing probability of tumor underdose with uncorrected rotations while rectal and bladder doses remained the same or reduced.25 Another study on CyberKnife using only translational corrections, showed significant reduction in D98 and D50 of PTV, although the absolute reduction was not clinically relevant. There was no significant impact on bladder/rectum/urethra dose volume histogram indices.26
Several MRI studies have correlated the AP intrafraction motion with rectal distension and peristalsis, although there seems to be minimal additional value over conventional tracking methods in determining PTV margins.27,28 In addition to motion tracking, use of an air-filled endorectal balloon or Foley catheter to immobilize the prostate have helped further reduce the positional uncertainty and PTV margins to * 2 mm, with variations within 1 mm for 95% of treatment sessions in the extreme hypofractionation study by Greco et al.29 Hydrogel spacers during CyberKnife treatment have also shown promise, especially over long treatment durations.30
Our dataset on intrafraction motion and deformation is among the largest reported datasets, and we conclude that there is differential motion, the highest being in longitudinal and lateral aspects, and the least in the AP aspect. Our study confines itself to the impact of intrafraction motion assessment after initial setup and correction of set-up error. Our findings of nearly similar motion in all 3 translational directions may be attributable to strict adherence to a rectal protocol as well as treating patients with an empty bladder such that AP motion seen in other studies was largely overcome. In contrast to the other literature reported on CyberKnife and other methods of tracking intrafraction motion, tracking and correction encompassed all 3 aspects of position variation (translation, rotation, deformation) and our margin recipe also included all of these.
Limitations of Fiducial-Based Tracking
Fiducial-based image-guided tracking in prostate SBRT carries several limitations. Fiducial implantation is an invasive procedure and may not be looked at favorably by patients and some physicians. Additionally, the tracking algorithm for CyberKnife, similar to the Calypso system, is best suited for rigid tumors. There is no option for tracking the movement and shape of adjacent critical structures, such as the bladder and rectum, which may also vary during treatment in addition to their relationship with the target (prostate). This, in turn, may alter the dose distribution and real-time irradiated volume during an RT fraction. Real-time tracking also substantially adds to treatment time, increasing the duration of a fraction to 30 to 45 minutes in contrast to 7 to 10 minutes for linac-based IMRT or VMAT. This increases the probability of prostate motion during the latter half of treatment, which is counterproductive.
Conclusions
Intrafraction motion and deformation are among the most important and challenging aspects of prostate external-beam radiation, and become even more relevant in the setting of dose escalation, hypofractionation, and SBRT due to the narrow therapeutic window. The interpatient, interfraction and intrafraction variability necessitate an individualized margin recipe for each image-guidance technique. We were able to achieve acceptable coverage for most patients despite the lower CTV to PTV margin of 3 mm in our study. Based on our findings, we suggest a differential margin of 5 mm in LR, and 4 mm in both craniocaudal and AP directions to ensure that at least 95% of the prescribed dose is delivered to at least 95% patients, thus maximizing the target coverage and minimizing the irradiated OAR volumes.
References
- Zietman AL, DeSilvio ML, Slater JD, et al. Comparison of conventional – dose vs high-dose conformal radiation therapy in clinically localized adenocarcinoma of the prostate: a randomized controlled trial. JAMA. 2005;294:1233-1239. doi:10.1001/jama.294.10.1233
- Pollack A, Zagars GK, Starkschall G, et al. Prostate cancer radiation dose response: results of the M.D. Anderson phase III randomized trial. Int J Radiat Oncol Biol Phys. 2002;53:1097-1105. doi:10.1016/s0360-3016(02)02829-8
- King CR, Lehmann J, Adler JR, et al. CyberKnife radiotherapy for localized prostate cancer: rationale and technical feasibility. Technol Cancer Res Treat. 2003;2:25-30. doi:10.1177/153303460300200104
- Chen Z, Yang Z, Wang J, et al. Dosimetric impact of different bladder and rectum filling during prostate cancer radiotherapy. Radiat Oncol. 2016;11:103.
- Wong JR, Grimm L, Uematsu M, et al. Image-guided radiotherapy for prostate cancer by CT-linear accelerator combination: prostate movements and dosimetric considerations. Int J Radiat Oncol Biol Phys. 2005;61:561-569. doi:10.1016/j.ijrobp.2004.06.010
- Chen J, Lee RJ, Handrahan D, et al. Intensity-modulated radiotherapy using implanted fiducial markers with daily portal imaging: assessment of prostate organ motion. Int J Radiat Oncol Biol Phys. 2007;68:912-919. doi:10.1016/j.ijrobp.2007.02.024
- Langen KM, Meeks SL, Poole DO, et al. The use of megavoltage CT (MVCT) images for dose recomputations. Phys Med Biol. 2005;50:4259-4276. doi:10.1088/0031-9155/50/18/002
- Litzenberg DW, Balter JM, Lam KL, et al. Retrospective analysis of prostate cancer patients with implanted gold markers using off-line and adaptive therapy protocols. Int J Radiat Oncol Biol Phys. 2005;63:123-133. doi:10.1016/j.ijrobp.2005.02.013
- Poulsen PR, Muren LP, Hoyer M. Residual set-up errors and margins in on-line image-guided prostate localization in radiotherapy. Radiother Oncol. 2007;85:201-206. doi:10.1016/j.radonc.2007.08.006
- Kupelian P, Willoughby T, Mahadevan A, et al. Multiinstitutional clinical experience with the Calypso System in localization and continuous, real-time monitoring of the prostate gland during external radiotherapy. Int J Radiat Oncol Biol Phys. 2007;67:1088-1098. doi:10.1016/j.ijrobp.2006.10.026
- Balter JM, Wright JN, Newell LJ, et al. Accuracy of a wireless localization system for radiotherapy. Int J Radiat Oncol Biol Phys. 2005;61:933-937. doi:10.1016/j. ijrobp.2004.11.009
- King CR, Brooks JD, Gill H, et al. Stereotactic body radiotherapy for localized prostate cancer: interim results of a prospective phase II clinical trial. Int J Radiat Oncol Biol Phys. 2009;73:1043-1048. doi:10.1016/j.ijrobp.2008.05.059
- Langen KM, Willoughby TR, Meeks SL, et al. Observations on real-time prostate gland motion using electromagnetic tracking. Int J Radiat Oncol Biol Phys. 2008;71:1084- 1090. doi:10.1016/j.ijrobp.2007.11.054
- Herschtal A, Kron T, Fox C. Radiotherapy margin design with particular consideration of high curvature CTVs. Med Phys. 2009;36:684-697. doi:10.1118/1.3070539
- Xie Y, Djajaputra D, King CR, et al. Intrafractional motion of the prostate during hypofractionated radiotherapy. Int J Radiat Oncol Biol Phys. 2008;72:236-246. doi:10.1016/j. ijrobp.2008.04.051
- Litzenberg DW, Balter JM, Hadley SW, et al. Influence of intrafraction motion on margins for prostate radiotherapy. Int J Radiat Oncol Biol Phys. 2006;65:548-553. doi:10.1016/j.ijrobp.2005.12.033
- Levin-Epstein R, Qiao-Guan G, Juarez JE, et al. Clinical assessment of prostate displacement and planning target volume margins for stereotactic body radiotherapy of prostate cancer. Front Oncol. 2020;10:539. doi:10.3389/fonc.2020.00539
- Kron T, Thomas J, Fox C, et al. Intrafraction prostate displacement in radiotherapy estimated from pre- and post-treatment imaging of patients with implanted fiducial markers. Radiother Oncol. 2010;95:191-197. doi:10.1016/j.radonc.2010.01.010
- Gottschalk AR, Hossain S, Chuang C, Descovich, Ma L. Intrafraction prostate motion during CyberKnife radiosurgery: implications on planning margins. Int J Radiat Oncol Biol Phys. 2008;72:S569. doi:10.1016/j.ijrobp.2008.06.147
- Yu H, Chen C, Tsai J, et al. Quantifying the accuracy of intrafractional internal target volume margin in stereotactic body radiotherapy for prostate cancer using CyberKnife Tumor Tracking System. Int J Radiat Oncol Biol Phys. 2011;81:874. doi:10.1016/j.ijrobp.2011.06.1561
- Shimizu S, Osaka Y, Shinohara N, et al. Use of implanted markers and interportal adjustment with real-time tracking radiotherapy system to reduce intrafraction prostate motion. Int J Radiat Oncol Biol Phys. 2011;81:393-399. doi:10.1016/j.ijrobp.2011.04.043
- Aubry JF, Beaulieu L, Girouard LM, et al. Measurements of intrafraction motion and interfraction and intrafraction rotation of prostate by three-dimensional analysis of daily portal imaging with radiopaque markers. Int J Radiat Oncol Biol Phys. 2004;60:30-39. doi:10.1016/j.ijrobp.2004.02.045
- Van de Water S, Valli L, Aluwini S, et al. Intrafraction prostate translations and rotations during hypofractionated robotic radiation surgery: dosimetric impact of correction strategies and margins. Int J Radiat Oncol Biol Phys. 2014;88:1154-1160. doi:10.1016/j.ijrobp.2013.12.045
- Wu Q, Ivaldi G, Liang J, et al. Geometric and dosimetric evaluations of an online image-guidance strategy for 3D-CRT of prostate cancer. Int J Radiat Oncol Biol Phys. 2006;64:1596-1609. doi:10.1016/j.ijrobp.2005.12.029
- Rijkhorst EJ, Lakeman A, Nijkamp J, et al. Strategies for online organ motion correction for intensity-modulated radiotherapy of prostate cancer: prostate, rectum, and bladder dose effects. Int J Radiat Oncol Biol Phys. 2009;75:1254-1260. doi:10.1016/j.ijrobp.2009.04.034
- Koike Y, Sumida I, Mizuno H, et al. Dosimetric impact of intrafraction prostate motion under a tumour-tracking system in hypofractionated robotic radiosurgery. PLoS One. 2018;13:0195296. doi:10.1371/journal.pone.0195296
- Padhani AR, Khoo VS, Suckling J, et al. Evaluating the effect of rectal distension and rectal movement on prostate gland position using cine MRI. Int J Radiat Oncol Biol Phys. 1999; 44:525-533. doi:10.1016/s0360-3016(99)00040-1
- Mah D, Freedman G, Milestone B, et al. Measurement of intrafractional prostate motion using magnetic resonance imaging. Int J Radiat Oncol Biol Phys. 2002;54:568-575. doi:10.1016/s0360-3016(02)03008-0
- Greco C, Pares O, Pimentel N, et al. Target motion mitigation promotes high-precision treatment planning and delivery of extreme hypofractionated prostate cancer radiotherapy: Results from a phase II study. Radiother Oncol. 2020;146:21-28. doi:10.1016/j.radonc.2020.01.029.
- Sumila M, Mack A, Schneider U, et al. Long-term intrafractional motion of the prostate using hydrogel spacer during Cyberknife treatment for prostate cancer – a case report. Radiat Oncol. 2014;9:186. doi:10.1186/1748-717X-9-186
Citation
Gupta D, Kaliyaperumal V, Bisht SS, Banerjee S, Goyal S, Narang K, Srivastava A, Mishra SR, Kataria T. Real-time Prostate Gland Motion and Deformation During CyberKnife Stereotactic Body Radiation Therapy. Appl Radiat Oncol. 2022;(1):21-31.
March 25, 2022