Planning Target Volume Margin Assessment of Retroperitoneal Tumors Using Robotic SBRT With Spine Tracking
Affiliations
- Department of Radiation Oncology, Brown Cancer Center, University of Louisville School of Medicine, Louisville, KY
- Central Arkansas Radiation Therapy Institute, Little Rock, AR
- School of Medicine, University of Louisville, Louisville, KY
Abstract
Objective:
Stereotactic body radiation therapy (SBRT) treatment is an emerging salvage modality for treating oligometastatic malignant lesions within the retroperitoneum. Appropriate planning target volume (PTV) margins are essential when delivering SBRT to effectively cover the target volume. Spine tracking uses bony spinal anatomy for localization during treatment delivery on robotic linear accelerator platforms. The aim of this study is to quantify the PTV margin needed when spine tracking is used for intrafraction motion tracking when treating retroperitoneal metastatic lesions with robotic SBRT.
Materials and Methods:
A single-institution chart review identified 16 patients with retroperitoneal tumors treated with SBRT over 19 courses in 103 fractions. Daily cone-beam CT images registered based on tumor positioning at the time of treatment were analyzed. Van Herk’s margin recipe was used to calculate the additional PTV margin required if spine tracking was used instead of daily tumor imaging. Patients’ tumors were stratified based on PTV proximity to the vertebral column (≤ 1 cm vs > 1 cm) and location within the retroperitoneum (superior vs inferior to renal artery), with descriptive statistics used to compare the differences of shifts based on location.
Results:
The additional margins calculated by Van Herk’s margin recipe to adequately cover the PTV within the 95% isodose surface for 90% of the entire patient cohort in the vertical, longitudinal, and lateral directions were 2.7, 2.8, and 2.8 mm, respectively. When tumors were stratified by proximity to the vertebral column, average longitudinal ( P < . 001) and total shifts ( P < . 001) were statistically significant.
Conclusion:
When treating retroperitoneal tumors with robotic SBRT, a minimum isometric margin expansion of 5 mm when creating the PTV is recommended if spine tracking is used for intrafraction motion assessment. Target volumes adjacent to the vertebral column may have PTV margins decreased to 4 mm without compromise in target coverage.
Introduction
Oligometastatic disease (OMD) is defined as a distinct cancer state in patients with a low metastatic disease burden in which a curative treatment is possible.1 A frequent location for OMD recurrence after local therapy for cancers of the lower abdomen and pelvis is the retroperitoneal lymph nodes. These nodal basins are comprised of the para-aortic, aortocaval, and paracaval lymph nodes.2 While some retroperitoneal recurrences are amenable to surgical salvage, many patients are either medically fragile and thus poor operative candidates or have a recurrent disease that is technically unresectable.
Stereotactic body radiation therapy (SBRT) has emerged as an attractive nonsurgical salvage option for recurrent tumors in the retroperitoneal region even in the setting of prior radiation therapy treatment.3 An increasing body of evidence suggests that incorporation of SBRT into metastasis-directed therapy of OMD improves patients’ oncological outcomes across a variety of histopathologies.4 - 9 Local control after SBRT in patients with limited OMD within the retroperitoneum has been estimated to be between 75% and 90% with favorable toxicity profiles.10 - 12
SBRT is predicated on administering large doses of radiation therapy in a limited number of fractions, resulting in a high biologically effective dose delivery to target tissues. In an effort to minimize normal tissue toxicity, SBRT attempts to achieve highly conformal dose distributions with rapid dose falloff.13 An important step in the treatment process for SBRT is the determination of margin size when expanding from either the gross target volume (GTV) or internal target volume (ITV) to the planning target volume (PTV). Appropriate setup margins should account for both systematic errors that influence all fractions of treatment, such as inaccuracies of mechanical equipment and photon beam dosimetry, and random errors such as daily patient setup changes that generally influence only a single fraction.14 If a chosen PTV margin is too small, the GTV or ITV will not fall within the prescription isodose line during the entire treatment delivery, which may underdose the target. Conversely, if a selected setup margin is made exceedingly large, more normal tissue will be unnecessarily irradiated.
SBRT may be delivered across a variety of radiation therapy treatment platforms with diverse image guidance capabilities. The CyberKnife (Accuray Inc.) is a robotic radiation therapy delivery platform that utilizes 2 ceiling-mounted kV x-ray imaging sources with corresponding in-floor image detectors positioned at 45° such that the generated beams intersect orthogonally at an imaging center.15 While the CyberKnife was initially designed as a radiosurgical platform, it is commonly used to treat extracranial sites of diseases, including tumors within the spine, lung, pancreas, liver, and prostate.16 - 20 Target tracking can be accomplished using the CyberKnife platform through multiple methods depending on the site of treatment; however, all utilize the 2 ceiling-mounted kV x-ray imaging sources. While bony anatomy is readily discernible with kV imaging, soft-tissue delineation is suboptimal, thus making direct target tracking unfeasible for many extracranial treatment sites. In place of direct target tracking, adjacent bony structures such as the spine may be used. With this method of tracking, the spine is monitored during treatment and delivery may be interrupted to allow for patient repositioning if necessary. Alternatively, fiducial tracking, which may be used with or without respiratory motion tracking, utilizes small radiopaque markers implanted within or near the tumor and serves as target surrogates identifiable by the kV x-ray imaging sources.
While multiple institutions have previously utilized robotic linear accelerator platforms to deliver SBRT to oligometastatic retroperitoneal lesions, significant variability exists across published literature with respect to treatment planning and delivery.21 - 26 While historically PTV margin determination has been largely institutionally defined, modern cooperative protocols investigating the use of SBRT for ablation of systemic OMD allow between 2 and 5 mm PTV margin additions depending on the site of the disease, immobilization technique used, and institutional setup accuracy.27 - 29 However, there are no prior reports guiding appropriate PTV margin selection when treating retroperitoneal tumors with robotic stereotactic radiation therapy. Thus, the aim of this study is to quantify the PTV margin needed when spine tracking is used for intrafraction motion tracking when treating retroperitoneal metastatic lesions with robotic SBRT.
Materials and Methods
A single-institution chart review was performed that identified consecutive patients > 18 years of age treated with linear accelerator-based SBRT for a single site of retroperitoneal OMD between 2015 and 2023. Patients with multiple courses of SBRT were included if they received treatment at an additional site of retroperitoneal disease > 6 months after their first course of treatment. The retroperitoneal lymph nodes were defined inferiorly at the level of the aortic or inferior vena caval bifurcation and superiorly to the diaphragmatic crura encompassing the para-aortic, aortocaval, and paracaval nodal basins. This study was determined to be exempted by the University of Louisville Institutional Review Board (IRB #22.0219).
To simulate the CyberKnife’s spine-tracking system, daily cone-beam CT (CBCT) images acquired using conventional linear accelerator onboard imaging systems at the time of SBRT treatment were rigidly registered to the treatment planning CT scan, aligned to the patient’s visible disease, and analyzed. Each CBCT was reviewed in Offline Review software (Aria, Varian). A region of interest, including the spine adjacent to the treatment volume but excluding as much soft tissue as possible, was delineated. Rigid registrations were reperformed within Offline Review using the region of interest and a bone intensity window and level so that the position of the spine on the CBCT was aligned to its position on the planning CT as close as possible to emulate the CyberKnife’s spine-tracking system. Per-patient and per-fraction shifts from the treatment position were recorded. Similar methodologies have previously been described and published by our group in a patient cohort receiving lung SBRT.30
Patients’ tumors were stratified and compared based on proximity to the vertebral column (≤ 1 cm vs > 1 cm) and location within the retroperitoneum (superior vs inferior location to the renal artery). Stratification of tumor location by proximity to the vertebral column using a distance cutoff of 1 cm was selected based on previously published phantom modeling evaluating the use of spine tracking for abdominal tumors showing smaller dose differentials and higher gamma analysis passing rates using acceptance criteria of dose difference and distance-to-agreement of 5%/5 mm at a distance of 1 cm from reference vertebrae.31 The renal artery was chosen to stratify superior vs inferior retroperitoneal tumor locations within the abdomen given its readily identifiable nature on CBCT imaging and was used as a surrogate for target proximity to the diaphragm. Tumors were considered superior to the renal artery if the treatment isocenter was superior to the last slice of either the left or right renal artery, whichever was more superior. Examples of the different types of tumor classifications are demonstrated in Figure 1 .
Examples of different tumor location classifications. Case 1: Planning target volume (PTV) proximity to the vertebral column > 1 cm and superior to the renal arteries in axial ( A ) and coronal views ( B ). Case 2: PTV proximity to the vertebral column ≤ 1 cm and inferior to the renal arteries in axial ( C ) and coronal views ( D ).
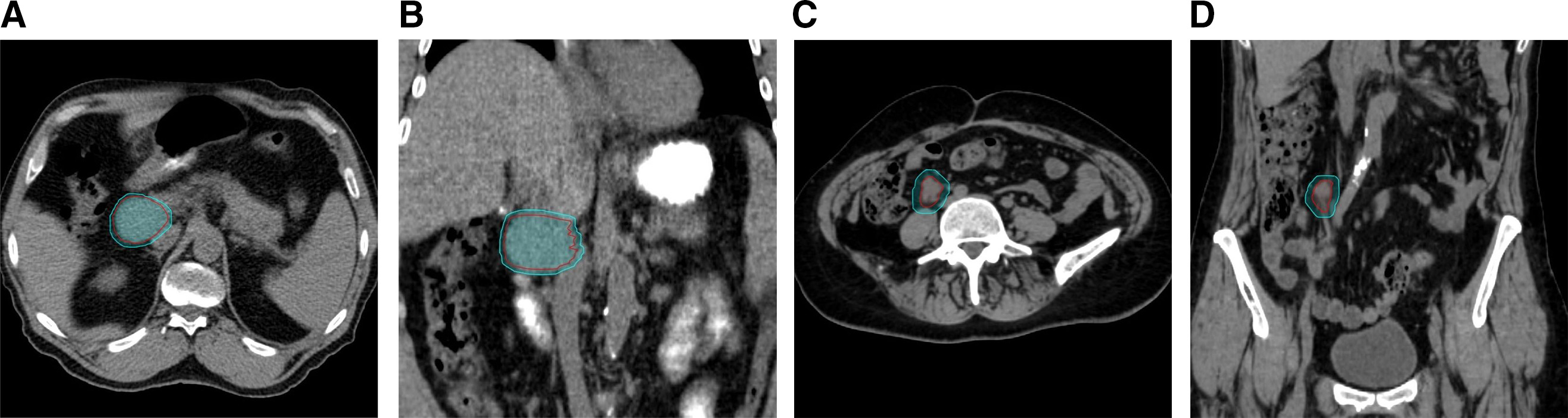
After chart review completion, per-patient mean shifts and standard deviations were used to calculate group systematic and random standard deviations. Using Van Herk’s margin recipe, additional margins that would adequately treat the patient population if spine tracking were used instead of direct daily tumor imaging by other image guidance techniques were obtained.32 , 33 Van Herk’s recipe calculates the additional margin in the vertical, longitudinal, and lateral directions for each of the tumor classifications using the following formula: 2.5∑ + 0.7σ, where ∑ is the group systematic standard deviation (standard deviation of the per-patient mean shifts) and σ is the group random standard deviation (standard deviation of the per-patient standard deviation of shifts). Formula coefficients of 2.5 and 0.7 were chosen such that the margin would adequately cover the GTV/ITV within the 95% three-dimensional isodose surface for 90% of the patient cohort. With this method, normal probability distributions were assumed for this patient cohort and different sources of error (eg, target delineation inaccuracies, setup error, organ motion) were inferred to be statistically independent. Student t -test was used to determine whether there was any statistical significance to the difference in shift values based on location. The significance level was set at P ≤ 0.02 in alignment with prior investigations by our group.30 Tumor size and absolute shift dimensions across all fractions were compared using the Pearson rank correlation coefficient. Statistical analysis and graphical illustrations were performed in Excel (Microsoft).
Results
Baseline patient characteristics are listed in Table 1 . A total of 16 patients with a single site of retroperitoneal OMD were treated over 19 courses in 103 fractions with SBRT between January 2015 and January 2023 and included for analysis. Genitourinary and gynecological histologies comprised a majority of the patient cohort (63%), with gastrointestinal (25%) and other (12%) histologies comprising a minority. Also, 7 treatment courses had PTVs of ≤ 15 cm3 (37%) compared with 4 with PTVs of 15-30 cm3 (21%) and 8 with PTVs of > 30 cm3 (42%), respectively. A total of 9 courses (48%) had PTV distances located ≤ 1 cm from the vertebral column compared with 10 (52%) > 1 cm. When stratified by tumor location within the retroperitoneum with respect to the renal arteries, 10 courses (52%) had superior retroperitoneal tumor classifications compared with 9 (48%) with inferior tumors. Motion management in the form of a four-dimensional CT (4DCT) scan obtained at the time of CT simulation and an ITV approach was utilized in a total of 12 courses (63%) vs 7 (37%) without. Of the 12 courses where a 4DCT was obtained, 8 were classified as a superior tumor location within the retroperitoneum in relation to the renal arteries, with mean tumor motion in the superior/inferior dimensions of 4.1 mm (range, 2.0-6.1 mm) vs a mean tumor motion of 1.9 mm (range, 0-3.0 mm) for lesions classified as located inferiorly within the retroperitoneum.
Patient Characteristics
n (%) | |
---|---|
Total patient cohort | 16 |
Treatment courses | 19 |
CBCT images | 103 |
Primary histology | |
GU/GYN | 10 (63%) |
GI | 4 (25%) |
Other | 2 (12%) |
PTV (cm3 ) | |
≤15 | 7 (37%) |
15-30 | 4 (21%) |
>30 | 8 (42%) |
Spine PTV distance (cm) | |
≤1 | 9 (48%) |
>1 | 10 (52%) |
Retroperitoneal location | |
Superior | 10 (52%) |
Inferior | 9 (48%) |
Motion management | |
Yes | 12 (63%) |
No | 7 (37%) |
Abbreviations: CBCT, cone-beam CT; GU/GYN, genitourinary/gynecological; GI, gastrointestinal; PTV, planning target volume.
The additional margins calculated by Van Herk’s margin recipe to adequately cover the PTV within the 95% isodose surface for 90% of the entire cohort in the vertical, longitudinal, and lateral directions were 2.7, 2.8, and 2.8 mm, respectively, and shown in Table 2 . The absolute shifts for CBCTs with the maximum total shift based on stratification between tumor location within the retroperitoneum (superior vs inferior) and PTV proximity to the vertebral column (≤ 1 cm vs > 1 cm) are listed in Tables 3 and 4 . Additional analysis was performed to determine the significance of tumor location within the retroperitoneum and proximity to the vertebral column on both unidirectional and total shifts. When stratified by proximity to the vertebral column, only the average longitudinal ( P < .001) and total shifts ( P < .001) were statistically significant and are highlighted in Table 4 . Further analysis was performed to identify any additional factors other than tumor location and proximity to the vertebral column that would affect shifts. The absolute shifts for each tumor were plotted as a function of PTV ( Figure 2 ). No correlation between the magnitude of the shift and PTV size was suggested ( R 2 = .0222).
Additional Planning Target Volume Margin Needed Across the Entire Patient Cohort
Vertical (mm) | Longitudinal (mm) | Lateral (mm) | |
---|---|---|---|
Group systematic standard deviation | .9 | .8 | .9 |
Group random standard deviation | .8 | 1.1 | .8 |
Additional margin | 2.7 | 2.8 | 2.8 |
Additional Margin (in mm) Needed When Stratified by Superior vs Inferior Retroperitoneal Classification Using Van Herk’s Margin Recipe With P Values Calculated Using Student t -Test
Classification | Vertical (mm) | Longitudinal (mm) | Lateral (mm) | Total Shift (mm) | |
---|---|---|---|---|---|
Group systematic standard deviation | Superior | .7 | .7 | .8 | .8 |
Inferior | 1.0 | 1.0 | 1.0 | 1.2 | |
Group random standard deviation | Superior | .7 | .9 | .7 | .8 |
Inferior | 1.0 | 1.3 | 1.0 | 1.2 | |
Additional margin | Superior | 2.2 | 2.3 | 2.4 | 2.5 |
Inferior | 3.2 | 3.4 | 3.3 | 3.9 | |
P values | - | .073 | .474 | .598 | .834 |
Additional Margin (in mm) Needed When Stratified by Proximity to Spine (≤ 1 cm vs > 1 cm) Using Van Herk’s Margin Recipe With P Values Calculated Using Student t -Test
Classification | Vertical (mm) | Longitudinal (mm) | Lateral (mm) | Total Shift (mm) | |
---|---|---|---|---|---|
Group systematic standard deviation | ≤1 cm | .6 | .5 | 1.0 | .6 |
>1 cm | .8 | .9 | .8 | 1.0 | |
Group random standard deviation | ≤1 cm | .5 | .8 | .9 | .8 |
>1 cm | .9 | 1.4 | .7 | 1.2 | |
Additional margin | ≤1 cm | 1.9 | 1.8 | 3.1 | 2.1 |
>1 cm | 2.7 | 3.3 | 2.5 | 3.4 | |
P values | - | .056 | <.001 | .340 | <.001 |
Correlation between tumor size and absolute shift. PTV, planning target volume.
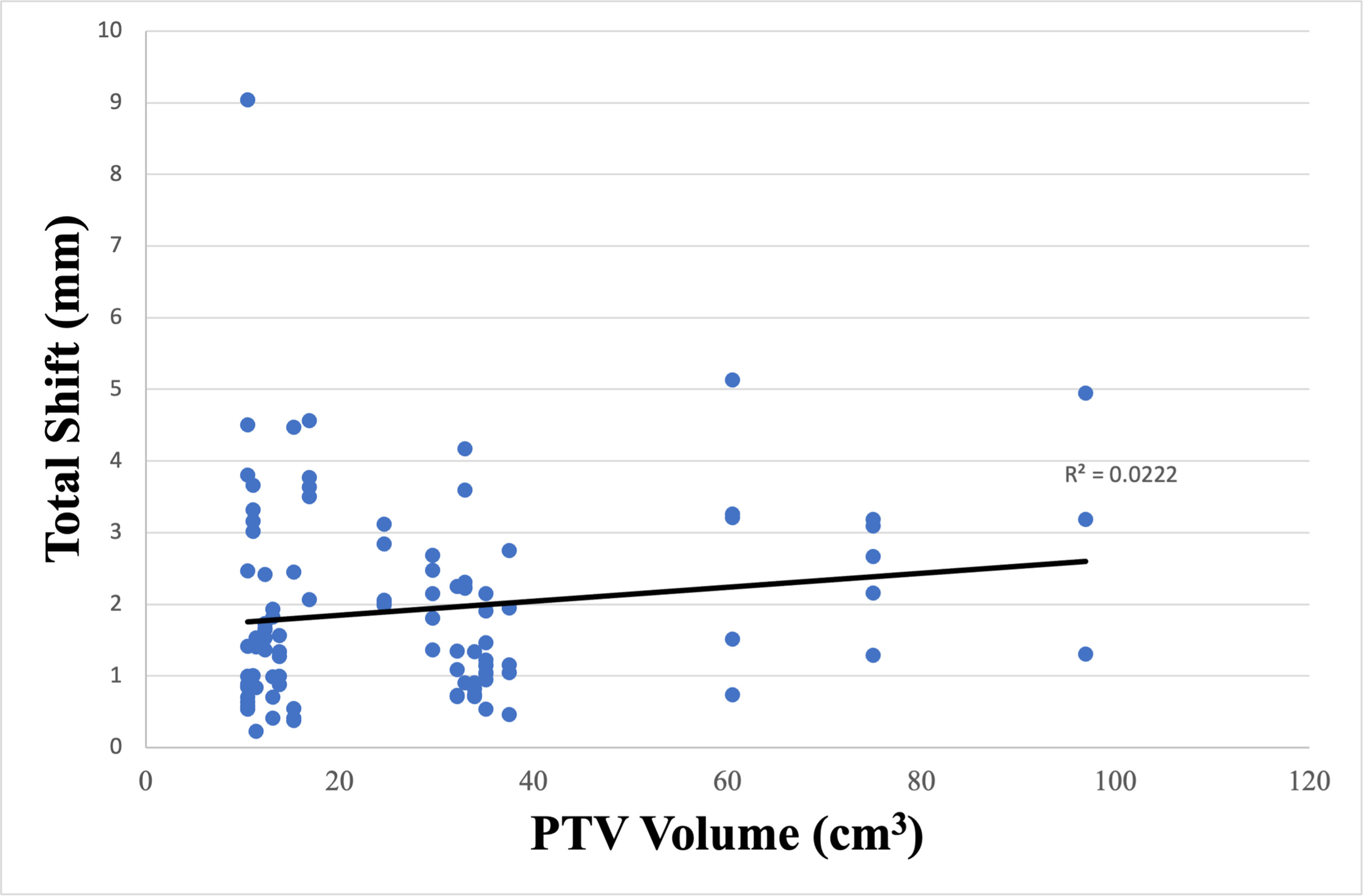
When stratified by location within the retroperitoneum, isometric PTV expansions of 3, 4, and 5 mm would have encompassed 82%, 94%, and 100% of the maximum total shifts for lesions superior to the renal artery vs 78%, 94%, and 98% for lesions inferior. Isometric PTV expansions of 3, 4, and 5 mm would have encompassed 55%, 76%, and 86% of the maximum total shifts for lesions > 1 cm from the vertebral column vs 94%, 100%, and 100% for lesions ≤ 1 cm and shown in Table 5 .
Isometric PTV Expansions Accounting for Maximum Total Shifts
Classification | 3 mm | 4 mm | 5 mm | |
---|---|---|---|---|
Location (superior vs inferior) | Superior (n = 49) | 40 (82%) | 46 (94%) | 49 (100%) |
Inferior (n = 54) | 42 (78%) | 51 (94%) | 53 (98%) | |
Spine PTV distance (≤ 1 cm vs > 1 cm) | ≤1 cm (n = 54) | 51 (94%) | 54 (100%) | 54 (100%) |
> 1 cm (n = 49) | 27 (55%) | 37 (76%) | 42 (86%) |
Abbreviation: PTV, planning target volume.
Discussion
When utilizing robotic radiation therapy delivery platforms to treat retroperitoneal tumors, target tracking may be performed using either fiducial marker tracking or spine tracking. Fiducial tracking within tumors enables sub-millimeter level of tracking accuracy for small target displacements and is often considered the gold standard for target tracking with robotic radiation therapy delivery platforms.34 However, fiducial marker implantation is not always feasible due to patient medical comorbidities or tumor anatomic location precluding safe implantation. Additional limitations of fiducial tracking are the requirement of at least 3 implanted fiducials to support 6 degrees of freedom (DOF) corrections and added procedural costs.35
Alternatively, intrafraction target tracking using surrogate structures, such as the spine, may be used. Spine tracking allows monitoring of a reference vertebra without the requirement of fiducial implantation using 6-DOF spatial information. A limitation of using spine tracking as a surrogate for the location of a retroperitoneal tumor is that it assumes the location of the tumor relative to the spine is constant from the time of simulation throughout the duration of treatment. However, treatment uncertainties may be introduced if the distance between the tumor and spine changes between simulation and treatment or if tumor motion patterns vary over time. As a result, an increased setup margin may be necessary, depending on the treatment site, to account for such sources of error. Selection of appropriately sized PTV margins is imperative when delivering high dose per fraction treatment with SBRT. When determining a PTV margin, radiation therapy departments must consider available image guidance within their clinic to ensure accurate dose delivery to target volumes.
Prior to this investigation, within our department, there was no standardized PTV margin addition when robotically treating retroperitoneal metastases with spine tracking. In this study, we found that minimum isometric PTV expansions of 3, 4, and 5 mm would have encompassed 55%, 76%, and 86% of the maximum total shifts for lesions >1 cm from the vertebral column vs 94%, 100%, and 100% for lesions ≤1 cm. Thus, our findings have informed our clinical PTV margin selection when treating with this modality for target lesions adjacent to the spine.
In the context of current literature, there is significant variability in treatment planning and delivery across institutions when treating retroperitoneal metastatic disease with robotic SBRT with spine tracking. Napieralska et al retrospectively reported the use of robotic SBRT using spine tracking to treat prostate cancer recurrences in 18 patients with a total of 31 metastatic lymph nodes located in the retroperitoneal region.26 Treatment doses and fractionation schedules varied, and an isometric GTV to PTV margin expansion of 5 and 4 mm was used in 28 and 3 lesions, respectively. Loi et al retrospectively reviewed 91 patients undergoing robotic SBRT for oligometastatic retroperitoneal failure without fiducial marker placement for pelvic, para-aortic, and upper abdominal lymph node failures.25 An isotropic PTV margin was used and varied between 2 and 5 mm around the GTV, with a median margin of 3 mm selected. Jereczek-Fossa et al analyzed 94 patients receiving robotic SBRT to 124 isolated prostate cancer lymph nodal recurrences in both pelvic and extra-pelvic lymph nodes using spine tracking without fiducial marker placement.21 A 2 mm margin was added to the GTV to obtain the PTV.
Limitations of this retrospective analysis include a small heterogeneous patient cohort treated at a single institution by multiple radiation oncologists over a period of 8 years. During this time, there was no institutionally defined PTV margin added to target volumes receiving SBRT to retroperitoneal sites nor were margins routinely isometric, introducing additional heterogeneity to this study cohort. Further, for purposes of this study, factors influencing group random and systemic errors were assumed to be constant across the entire patient cohort over time. Van Herk’s margin recipe assumes the sample population and sources of introduced error are normally distributed across a given study population.32 However, patient setup errors may not follow a normal distribution if collected over a short period of time in a small sample size.36
Additionally, motion management was only assessed in a total of 12 treatment courses (63%) of our cohort. It has been reported that the diaphragmatic motion of abdominal lesions may reach 40 mm even during shallow respiration cycles.37 Van Herk’s margin recipe attempts to calculate the necessary additional PTV margin to ensure adequate dose coverage to a defined clinical target volume (CTV), which includes both gross disease in addition to subclinical microscopic disease. When utilizing SBRT, the generation of a CTV from a GTV or ITV is often omitted. Additionally, when using a 4DCT to generate an ITV, internal target motion is accounted for within treatment volumes, thus reducing the potential source of both systematic and random errors. Within this study population, a 4DCT/ITV approach was utilized in a total of 12 of 19 courses when delivering SBRT. At our institution, when treating extrathoracic sites of disease with SBRT, use of 4DCT imaging at the time of CT simulation is left to the discretion of the treating radiation oncologist. Thus, a large portion of this study cohort without known internal target motion may underestimate this potential source of error. However, even when using a 4DCT, further treatment uncertainties may arise from interfraction and intrafraction changes of organ motion, motion from unpredictable respiratory cycles, as well as variations between the imaging and treatment sessions.
We identified no significant differences in unidirectional and total shifts when tumors were stratified based on superior or inferior location to the renal arteries. The renal artery was chosen to stratify superior vs inferior retroperitoneal tumor locations within the abdomen given its readily identifiable nature on CBCT imaging. However, this is an unvalidated surrogate for target proximity to the diaphragm and respiratory cycle tumor motion. Within the contexts of this study, use of this landmark for such purposes should be viewed as hypothesis generating and requires additional validation.
Chan et al suggested that when robotic SBRT for lung cancers is delivered with spine tracking, unless effective means are employed to reduce tumor motion, caution should be undertaken when treating tumors with motion of more than 10 mm due to temporal dose variations from considerable intrafractional target motion.38 Technical discussions regarding Accuray’s Xsight Spine Tracking system utilized by our clinic are beyond the scope of this report but are explained further in detail by Ho et al.39 Future directions of study for this topic by our group include analyzing dosimetric variables for both organs at risk (OARs) and targets when utilizing the additional calculated Van Herk’s margins when treating retroperitoneal sites of disease with robotic SBRT.
Lastly, clinical judgment should be deferred to the treating physician for PTV margin selection in close collaboration with medical physics and dosimetry and performed on a per-patient basis accounting for the size of the treated lesion and proximity to OARs. Nonisometric PTV expansions are also appropriate and frequently used in clinical practice, which varies based on the treating institution. When selecting a setup margin, clinicians must weigh the likelihood of increased treatment-related morbidity from higher integral dose to adjacent normal structures for larger setup margins vs potential target underdosing if smaller margins are used.
Conclusions
When treating retroperitoneal tumors with robotic SBRT, an isometric PTV margin expansion of 5 mm is recommended if spine tracking is used for intrafraction motion assessment. Target volumes located near the vertebral column may have PTV margins decreased to 4 mm without compromising target coverage. Additional factors such as target motion, dose per fraction size, and institutional quality assurance should be considered for patient-specific PTV margin expansions.
References
Citation
Grant McKenzie, MD, Maxwell Kassel, BS, Andres Portocarrero Bonifaz, MS, Andrew Willett, BS, Christine Swanson, PhD, Joshua James, MS, Neal Dunlap, MD. Planning Target Volume Margin Assessment of Retroperitoneal Tumors Using Robotic SBRT With Spine Tracking. Appl Radiat Oncol. 2024;(1):30 - 38.
doi:10.37549/ARO-D-23-00029
March 1, 2024