Actualizing Risk-Adapted Thoracic Stereotactic Body Radiation Therapy with MR Guidance
Images
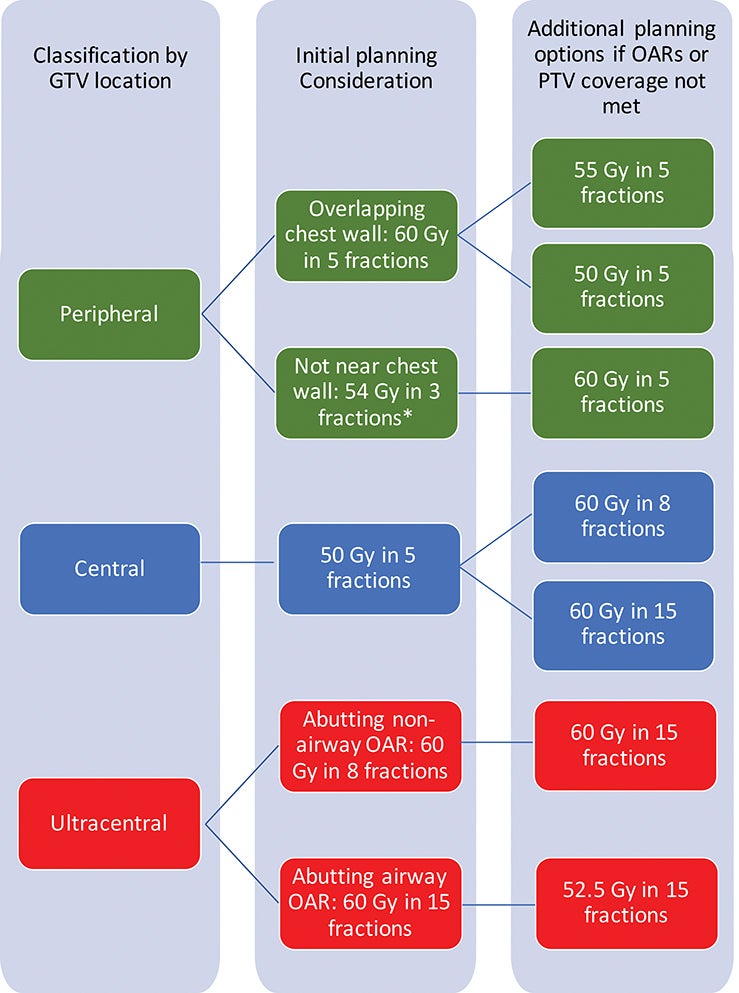
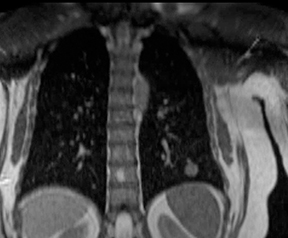
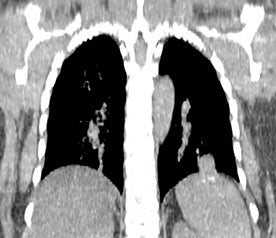
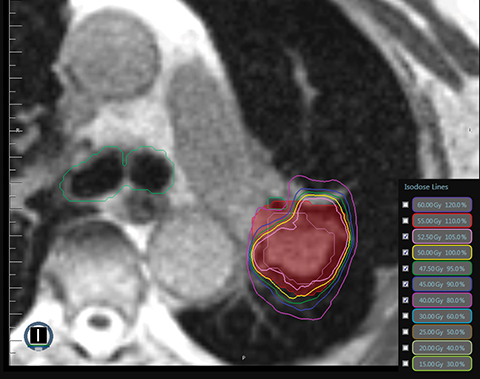
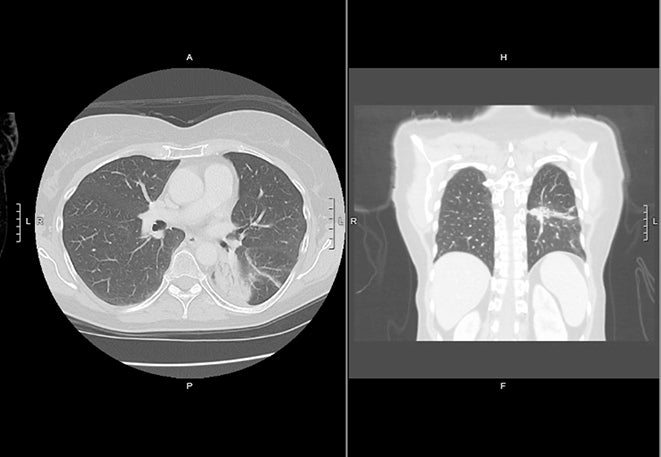
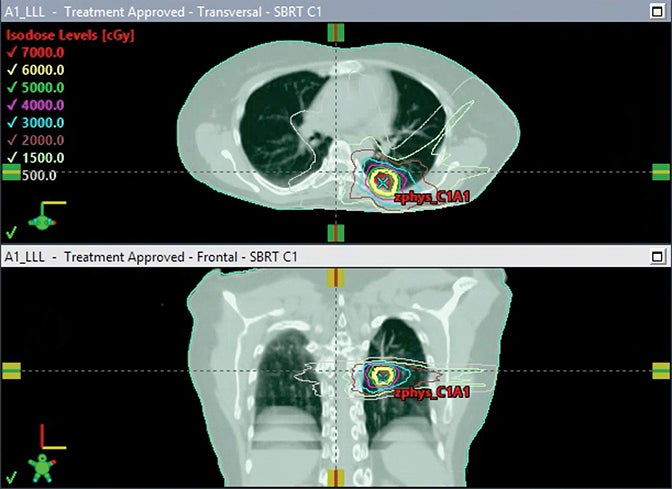
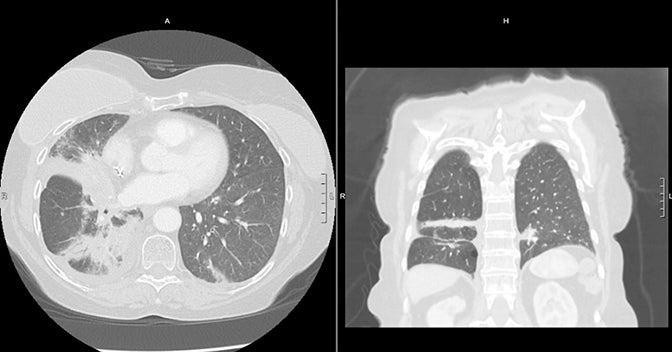
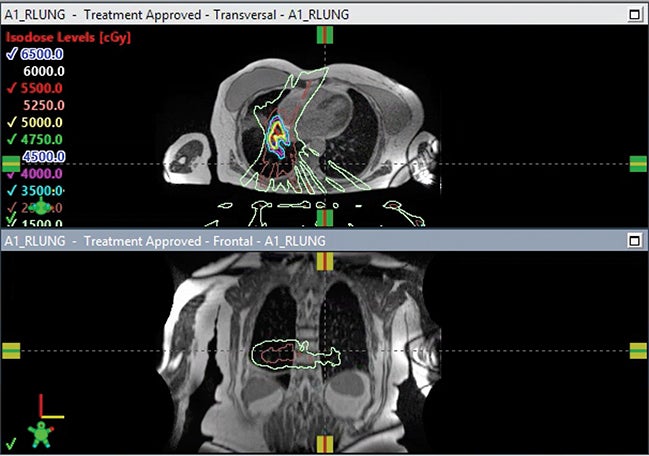
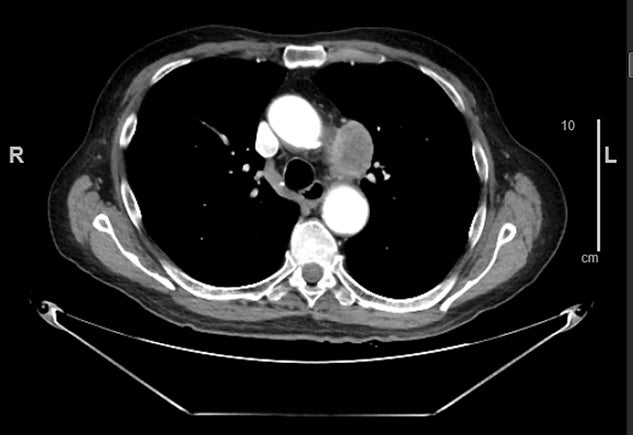
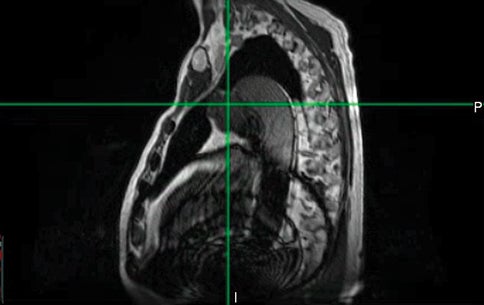
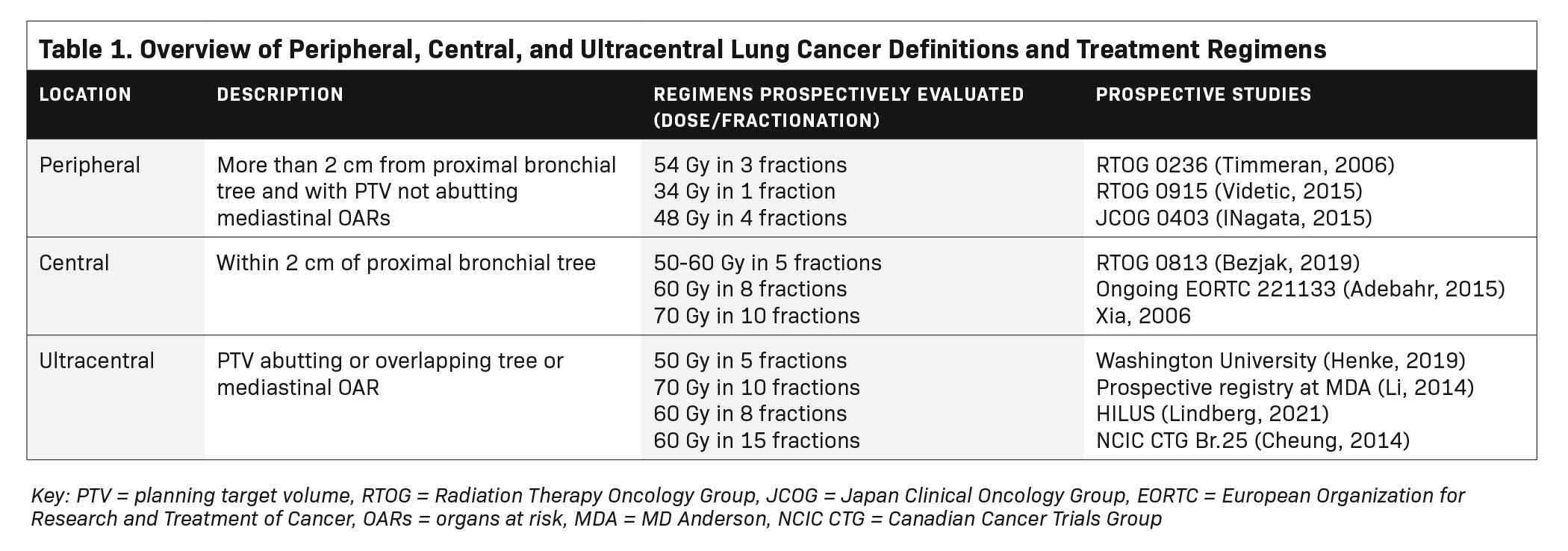
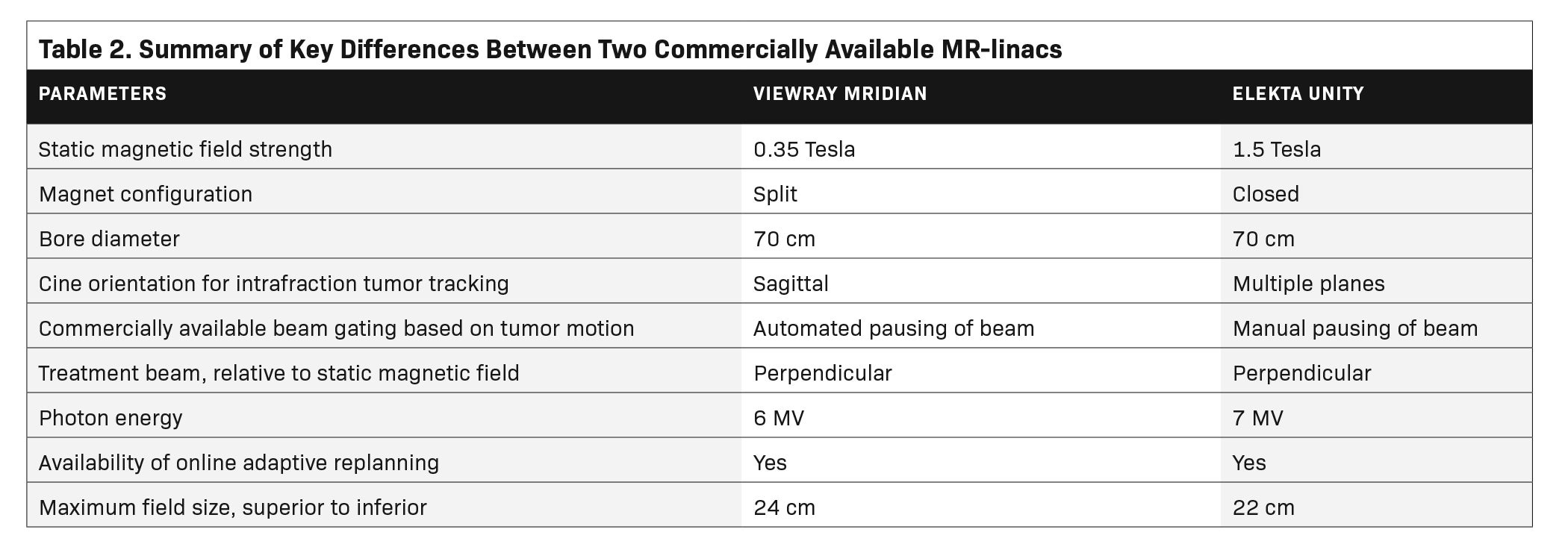
SA-CME credits are available for this article here.
Lung cancer is the leading cause of cancer death in both men and women in the US.1 Although most lung cancers are stage IV at the time of diagnosis,2 lung cancer screening enables the diagnosis of earlier lesions and reduces mortality.3 Thoracic stereotactic body radiation therapy (SBRT) is a noninvasive alternative to surgery for patients with early stage lung cancers, and its use is supported by multiple studies including the randomized trial CHISEL, which compared SBRT to radiation therapy delivered with standard fractionation and demonstrated improved local control with SBRT.4 Based on available data to date, 5-year tumor control with SBRT is considered around 90%.5,6
Randomized data comparing surgery with SBRT is more limited. Two randomized trials, STARS and ROSEL, which compared lobectomy to SBRT, were closed early due to slow accrual. A secondary unplanned combined analysis showed excellent outcomes with SBRT, with 3-year overall survival (OS) of 95%, 3-year recurrence-free survival of 86%, and grade 3 toxicity of 10%, with no grade 4 or 5 toxicities.7 After expansion of the SBRT arm of the STARS trial to a single-arm study with longer follow-up, 5-year OS was 87% and 5-year progression free survival (PFS) was 77%, comparing favorably to a matched cohort of patients treated with surgery, with no differences in PFS or cumulative incidence of local, regional, or distant failures. Three- and 5-year OS were higher with SBRT.8 Given the caveats of these analyses, further data are needed. Additional trials including the randomized studies STABLE-MATES (NCT02468024) and VALOR (NCT02984761) are underway.
Despite the excellent local control seen with SBRT, severe toxicities have been reported in tumors near the airways and mediastinal structures, leading to classification of tumors as peripheral (noncentral), or central (within 2 cm of the proximal bronchial tree including the distal 2 cm of the trachea and lobar bronchi).9 Within central tumors, ultracentral has emerged as a higher risk category of tumors.10 While the definition of ultracentral has differed across studies, ultracentral generally refers to abutment of the tumor or planning target volume (PTV) with critical organs at risk (OARs) such as the proximal bronchial tree, esophagus, or great vessels. In the SUNSET trial, ultracentral tumors were defined as those with a PTV touching or overlapping the central bronchial tree, esophagus, pulmonary vein or pulmonary artery.10 Various SBRT regimens have been tested prospectively for peripheral,9,11,12 central,13-15 and ultracentral16-19 lung tumors. These classifications and regimens are summarized in Table 1.
Reports of excess toxicity for tumors in high-risk locations have also led to risk-adapted approaches to SBRT, which balance tumor coverage at adequate biologic effective dose for alpha/beta of 10 (BED10) with OAR sparing. An example of an institutional approach using a conventional linear accelerator is shown in Figure 1. In this example, if OARs cannot be spared without compromising tumor coverage, further fractionation is pursued. Based on data from Onishi et al,20 local control and OS are inferior below a BED10 of 100; thus, in the example approach in Figure 1, rather than compromising adequate tumor coverage to meet OARs, alternate regimens are considered to maximize BED10 to the extent possible.
The risk-adapted approach shown in Figure 1 is impacted by the additional options afforded by MR-linacs. An MR-linac is a device combining an MRI scanner with a linear accelerator, currently available as FDA-approved devices from Elekta, as the Unity, and from ViewRay, as the MRIdian.21 MR-linacs allow for MR-guided tumor setup and real-time MRI monitoring during treatment for tumor tracking. Importantly, these technologies permit online adaptive radiation therapy, the replanning of radiation treatment with the patient on the treatment table, accounting for daily differences in tumor and OAR location and morphology. Online adaptive radiation therapy is also possible on non-MR-linacs through systems such as Ethos (Varian).22 OARs contoured for initial and adaptive MR-linac plans are similar to OARs contoured for conventional non-MR guided, nonadaptive radiation therapy.23 In the absence of new data, dose constraints for MR-guided SBRT are generally based on accepted constraints used for non-MR linac SBRT. Due to the dose fall-off with SBRT and time required for recontouring, MR-guided online adaptive recontouring may focus on the recontouring of structures within a high-dose region (2 to 3 cm from the target) on treatment day. Outside of this high-dose region, the dose is unlikely to violate OAR metrics. When delivered using an MR-linac, adaptive SBRT is often referred to as stereotactic MR-guided adaptive radiation therapy (SMART), with various workflows previously described.24,25 SMART is available for both Elekta26 and ViewRay27 MR-linacs. Although the specific steps for SMART differ by vendor, SMART requires steps to be implemented on treatment day including acquisition of a new MRI on the day of treatment, recontouring on the MRI acquired at treatment, plan reoptimization, plan approval, and quality assurance checks prior to delivery. Lung lesions have been treated with both systems.28,29 Major differences between the Elekta Unity and ViewRay MRIdian MR-linacs are shown in Table 2.30-33 Currently, there is no consensus on clinical indications for utilizing an adaptive replan; thresholds for requiring SMART vary by center and disease site. MR-linacs can be used to treat lung cancer in peripheral, central, and ultracentral locations, with relevant data and considerations reviewed. Due to the ability of SMART to account for daily changes in target and OARs, and the potential for reduced PTV margins with real-time MR guidance, SMART may also be beneficial for thoracic reirradiation in these locations.
Peripheral Lesions
Peripheral thoracic tumors are more than 2 cm from the proximal bronchial tree, not involving the central pleura or mediastinum. They can be further classified by their proximity to the chest wall. Chest wall proximity is important to recognize due to the association of chest wall dose with severe chest wall pain and rib fracture.34 V30 < 30 cc is a constraint used for 3-fraction regimens.34 Optimized V37, the 5-fraction BED3 equivalent of V30, was associated with reduced toxicity in a retrospective study of 5-fraction regimens.35
Safety and efficacy of single-fraction SBRT for peripheral tumors was demonstrated in the prospective trial RTOG 0915.11,36 In data from Cleveland Clinic, which included a review of patients treated on RTOG 0915, chest wall toxicity with single-fraction regimens was increased with abutment or proximity of the chest wall, with grade 2 to 4 toxicity 5.7% in tumors >2 cm from the chest wall and 30.6% for tumors abutting the chest wall.37 Proximity to the chest wall or to central structures remains a challenge for the safe implementation of single-fraction regimens in peripheral tumors.
Peripheral tumors close to the diaphragm may also experience significant respiratory motion, leading to larger volume internal target volumes (ITVs) when motion reduction techniques are not used. Use of breath-hold gating, which has also been performed on conventional linacs, can mitigate this effect. The availability of MR imaging during treatment for real-time breath hold monitoring may be an advantage of MR-guided SBRT for the treatment of peripheral tumors susceptible to motion (Figure 2A-2B). In addition, the daily adaptive replanning enabled by MR-linac treatment may be useful for rapidly growing, or shrinking, tumors abutting the chest wall due to the ability of adaptive replanning to account for daily changes in the relationship between tumor position and chest wall. An important consideration for the treatment of peripheral tumors with SMART is that arms are typically positioned at the sides due to the time on table. An arms-down position may limit beam angles and may also bring the inframammary fold into the field.
In a report of 23 patients with peripheral lung tumors treated with SMART in the Netherlands, online adaptation improved PTV coverage.38 For the subset of patients who also underwent free-breathing 4-dimensional computed tomography (4DCT), SMART breath-hold PTVs were 53.7% the volume of PTVs generated from an ITV. In a subsequent study of 17 patients simulated at the same center for planned single-fraction MR-guided SBRT,39 7 patients were unable to undergo single-fraction SBRT (because of difficulty in gross tumor volume [GTV] tracking due to tumor size in 1 patient, difficulty in GTV tracking due to blood vessels in 4, proximity to chest wall in 1 patient, and difficulty in breath holds in 1 patient). Of the 10 undergoing single-fraction SBRT, 9 completed treatment in one session, and median total in-room procedural time was 120 minutes. Online adaptive replanning improved PTV coverage but did not impact GTV coverage. These studies provide support for the use of SMART in peripheral tumors. The phase I trial SMART ONE (NCT04939246) is assessing the feasibility of single-fraction SMART in tumors in the lung as well as other sites.
Due to the limited availability of simulation appointments at many centers, and the difference in immobilization required for MR-guided vs non-MR-guided simulation, additional research is also needed to better identify optimal candidates for MR-guided RT. A recent case report, which did not require online adaptive replanning, noted a reduced treatment time for single-fraction lung SBRT.40 The ability to better predict which tumors do not benefit from online adaptive replanning could also further reduce on-table time.
Central Lesions
Central tumors are within 2 cm of the proximal bronchial tree, with further subclassification of tumors touching the tree as ultracentral. Due to the proximity of the proximal bronchial tree, these tumors are at increased risk for OAR toxicity as seen on the phase II prospective study reported by Timmerman et al.9 The online adaptive replanning workflows utilized in MR-guided SBRT may be promising for central tumors due to the ability to account for daily changes in OAR location and morphology. In addition, MR-linacs allow for real-time tumor tracking during treatment, which may be helpful in decreasing appropriate PTV margin. A decreased PTV margin could enable improved target coverage while sparing OARs.
In a retrospective analysis from VU Medical Center in the Netherlands of 25 patients with central lung tumors treated with SMART in 5 or 8 fractions, the reoptimized plan was selected in 92% of cases.41 SMART also reduced the number of OAR violations. The feasibility of SMART for central lung tumors is being studied on a prospective, phase I trial in the US (NCT04115254). The prospective phase II trial LUNG STAAR (NCT04917224) is assessing SMART in central lung cancers.
Ultracentral Lesions
Although ultracentral tumors have been defined variably across studies, definitions generally consider these tumors as touching, close to (< 1 cm) or overlapping critical OARs. The SUNSET trial categorized PTVs abutting the tree or mediastinal OARs as ultracentral.10 SBRT for ultracentral tumors requires careful consideration of tumor location and motion. Retrospective data from Memorial Sloan Kettering Cancer Center identified an increased risk of grade 5 toxicity (hazard ratio 16.9, confidence interval 3.2-88%) in ultracentral tumors treated with SBRT in the setting of antiangiogenic agents. The recent prospective HILUS trial reported grade 5 toxicity in 10 of 65 patients (15%) using a regimen of 60 Gy in 8 fractions, with dose to the hottest 0.2 cc of main bronchi and trachea as the strongest predictor for grade 5 bronchopulmonary hemorrhage.42 In this trial, dose was prescribed to the 67% isodose line, with hot spots of 150%. The protocol did not specify OAR dose guidelines for the lobar and segmental bronchi.
MR-guided SBRT holds promise for ultracentral tumors due to the potential for MR-guided setup and tumor tracking with real-time MRI during treatment, as well as the potential for online adaptive replanning to ensure sparing of OARs based on daily geometry and morphology (Figure 2C). Online adaptive replanning can also ensure that absolute dose constraints are met daily. In a prospective feasibility study from Washington University in St. Louis, 5 patients with ultracentral tumors were treated with 50 Gy in 5 fractions, with 10 of 25 fractions requiring adaptive replanning to improve PTV coverage or reverse OAR violations.16 Case reports and case series describe the safety and feasibility of MR-guided SBRT for cardiac lesions, which are ultracentral by definition due to their location in the heart.43-46
Challenges for MR-guided SBRT for ultracentral tumors include identification and contouring of structures that may be better defined on CT such as the proximal bronchial tree, the limited spatial resolution of MR and challenge in tracking very small tumors, and the use of IMRT on MR-linacs, which may have a less favorable distribution of low dose compared to VMAT. In Figure 3, a case of CTCAE (Common Terminology Criteria for Adverse Events) grade 1 (asymptomatic) pneumonitis is shown for a patient treated with SBRT on a non-MR linac using volumetric-modulated arc therapy (VMAT). A case of CTCAE grade 1 pneumonitis is also shown for a patient with an ultracentral tumor treated with MR-guided SBRT using static field, coplanar (intensity-modulated radiation therapy) IMRT on an MR-linac. The low dose is more diffuse in the MR-linac case, leading to radiation changes even in the contralateral lung.
Despite the potential of MR-guided SBRT for the treatment of peripheral, central, and ultracentral primary and secondary lung cancers, challenges to the broad application of this technique remain. Evidence to date has largely been in the form of retrospective or prospective phase I trials, motivating the need for additional phase II or III data. In addition, patients with primary lung cancer may have comorbidities such as chronic obstructive pulmonary disease, limiting ability to breath-hold or to lay flat for the 1 hour or more duration of SMART. Careful assessment of patients prior to simulation is needed. MR-unsafe pacemakers or other devices may preclude the use of MR-guided SBRT for some patients. Artifacts can also be associated with MR conditional implants, impairing the ability to contour or safely track. For example, in Figure 4, the sagittal cine obtained at MR simulation is shown for a patient with an ultracentral lung metastasis and an MR conditional aortic stent. The artifact from the stent was inferior to the target, but cosagittal, and precluded safe tracking on sagittal cine during treatment. This patient was treated with non-MR-guided SBRT with an excellent response. This case illustrates the need for both MR-guided and non-MR-guided SBRT options for patients.
Future Directions
In light of the toxicity seen on HILUS and other studies, additional research is needed to ensure the safe and effective treatment of central and ultracentral lung cancers. Use of radiosensitizers could improve the therapeutic window by allowing for the utilization of lower radiation treatment doses, which when combined with a radiosensitizer localizing to tumor, could allow for simultaneous local control of the tumor and improved normal tissue sparing. Given the promise of MR-guided radiation therapy for the treatment of central and ultracentral lung tumors, gadolinium-based nanoparticles may be especially useful due to their ability to localize the tumor and serve as radiosensitizers. Such agents that provide both therapeutic and diagnostic information are also known as theranostics.47
The Nano-Rad study in France recently demonstrated the safety of combining gadolinium-based nanoparticles with whole-brain radiation in patients with multiple brain metastases.48 The selective distribution of the nanoparticle in brain metastases was also demonstrated. A similar preferential distribution in lung tumors would be particularly beneficial for the treatment of central and ultracentral cancers in close proximity to normal tissues if doses lower than standard ablative SBRT doses could be utilized. A clinical trial is currently open studying the use of gadolinium-based nanoparticles in the MR-guided treatment of central lung cancers (NCT04789486). Because the clinical efficacy of gadolinium-based nanoparticles in this setting is unknown, the trial utilizes an ablative prescription dose of 50 Gy in 5 fractions. In the future, it would be particularly advantageous for ultracentral tumors if radiosensitizers could enable treatment of these cancers to doses of 40 Gy in 5 fractions, or lower, facilitating sparing of more sensitive normal tissues.
Artificial intelligence (AI) may help accelerate efficiency and facilitate the adoption of MR-guided radiation therapy. Currently, there is a daily, time-intensive process of manual recontouring required for daily online adaptive replanning. Due to the proximity of nearby normal tissues, central, ultracentral, and peripheral lung tumors frequently require recontouring of dose-limiting OARs. AI algorithms, including deep learning, have shown the ability to produce highly accurate OARs within seconds on both CT and MR images.49,50 AI has also shown promise in tumor auto-contouring, which can be coupled with online dose optimization to limit time for adaptive replanning.51 Because radiation therapists, radiographers, and physicists also participate in recontouring at some centers,52,53 AI has the potential to improve workflows for the interdisciplinary team. As SBRT options for patients broaden, AI may also be able to accelerate development of mock plans,54 allowing for comparison plans to be generated proactively, assisting in selection of fractionation scheme and MR-linac vs non-MR linac for each patient. This will allow for truly individualized, risk-adaptive SBRT.
Conclusions
MR-linacs expand options for the treatment of thoracic tumors with SBRT by offering excellent soft-tissue contrast compared with CT, as well as additional advantages including MR-guided setup, online adaptive replanning, and real-time MRI-based monitoring of breath-hold treatments. For peripheral tumors, MR-guided SBRT may be advantageous in minimizing dose to the chest wall, and in minimizing normal lung treated with MR-guided breath-hold treatment. MR-guided setup may help with the visualization and sparing of OARs in the treatment of central and ultracentral tumors with SBRT. Disadvantages of MR-guided SBRT for peripheral, central, and ultracentral lung tumors include the potential for longer times on the treatment table and incompatibility of this technology with MR unsafe implants. Future work evaluating MR-guided lung SBRT on prospective studies is needed. By allowing OAR sparing with the potential for dose escalation, MR-guidance holds promise in improving risk-adapted SBRT for the treatment of thoracic malignancies.
References
- Siegel RL, Miller KD, Jemal A. Cancer statistics, 2020. CA Cancer J Clin. 2020;70(1):7-30. doi:10.3322/caac.21590
- Cancer of the lung and bronchus – cancer stat facts. NIH National Cancer Institute Surveillance, Epidemiology, and End Results Program. Accessed June 6, 2021. https://seer.cancer.gov/statfacts/html/lungb.html
- Sands J, Tammemägi MC, Couraud S, et al. Lung screening benefits and challenges: a review of the data and outline for implementation. J Thorac Oncol. 2021;16(1):37-53. doi:10.1016/j.jtho.2020.10.127
- Ball D, Mai GT, Vinod S, et al. Stereotactic ablative radiotherapy versus standard radiotherapy in stage 1 non-small-cell lung cancer (TROG 09.02 CHISEL): a phase 3, open-label, randomised controlled trial. Lancet Oncol. 2019;20(4):494-503. doi:10.1016/S1470-2045(18)30896-9
- >Timmerman RD, Hu C, Michalski J, et al. Long-term results of RTOG 0236: A phase II trial of stereotactic body radiation therapy (SBRT) in the treatment of patients with medically inoperable stage I non-small cell lung cancer. Rad Oncol Biol. 2014;90(Supplement):S30. doi:10.1016/j.ijrobp.2014.05.135
- Senthi S, Lagerwaard FJ, Haasbeek CJA, Slotman BJ, Senan S. Patterns of disease recurrence after stereotactic ablative radiotherapy for early stage non-small-cell lung cancer: a retrospective analysis. Lancet Oncol. 2012;13(8):802-809. doi:10.1016/S1470-2045(12)70242-5
- Chang JY, Senan S, Paul MA, et al. Stereotactic ablative radiotherapy versus lobectomy for operable stage I non-small-cell lung cancer: a pooled analysis of two randomised trials. Lancet Oncol. 2015;16(6):630-637. doi:10.1016/S1470-2045(15)70168-3
- Chang JY, Mehran RJ, Feng L, et al. Stereotactic ablative radiotherapy in operable stage I NSCLC patients: long-term results of the expanded STARS clinical trial. J Clin Oncol. 2021;39(15 supplement):Abstract8506. doi:10.1200/JCO.2021.39.15_suppl.8506
- Timmerman R, McGarry R, Yiannoutsos C, et al. Excessive toxicity when treating central tumors in a phase II study of stereotactic body radiation therapy for medically inoperable early-stage lung cancer. J Clin Oncol. 2006;24(30):4833-4839. doi:10.1200/JCO.2006.07.5937
- Giuliani M, Mathew AS, Bahig H, et al. SUNSET: Stereotactic Radiation for Ultracentral Non-Small-Cell Lung Cancer – Safety and Efficacy Trial. CLLC. 2018;19(4):e529-e532. doi:10.1016/j.cllc.2018.04.001
- Videtic GMM, Hu C, Singh AK, et al. A randomized phase 2 study comparing 2 stereotactic body radiation therapy schedules for medically inoperable patients with stage i peripheral non-small cell lung cancer: NRG Oncology RTOG 0915 (NCCTG N0927). Int J Radiat Oncol Biol Phys. 2015;93(4):757-764. doi:10.1016/j.ijrobp.2015.07.2260
- Nagata Y, Hiraoka M, Shibata T, et al. A phase II trial of stereotactic body radiation therapy for operable T1N0M0 non-small cell lung cancer; Japan Clinical Oncology Group (JCOG0403); long term follow-up results. Radiat Oncol Biol. 2018;102(3):S9. doi:10.1016/j.ijrobp.2018.06.115.
- Safety and efficacy of a five-fraction stereotactic body radiotherapy schedule for centrally located non–small-cell lung cancer: NRG Oncology/RTOG 0813 Trial. J Clin Oncol. April 2019:JCO.18.00622–15. doi:10.1200/JCO.18.00622
- Adebahr S, Collette S, Shash E, et al. LungTech, an EORTC Phase II trial of stereotactic body radiotherapy for centrally located lung tumours: a clinical perspective. BJR. 2015;88(1051):20150036. doi:10.1259/bjr.20150036
- Xia T, Li H, Sun Q, et al. Promising clinical outcome of stereotactic body radiation therapy for patients with inoperable stage I/II non-small-cell lung cancer. Radiation Oncology Biology. 2006;66(1):117-125. doi:10.1016/j.ijrobp.2006.04.013
- Henke L, Olsen JR, Contreras JA, et al. Stereotactic MR-guided online adaptive radiation therapy (SMART) for ultracentral thorax malignancies: results of a phase 1 trial. 2019;4(1):201-209. doi:10.1016/j.adro.2018.10.003
- Li Q, Swanick CW, Allen PK, Gomez DR, Welsh JW, Liao Z. Stereotactic ablative radiotherapy (SABR) using 70Gy in 10 fractions for non-small cell lung cancer: Exploration of clinical indications. Radiother Oncol. 2014;112(2):256-261. doi:10.1016/j.radonc.2014.07.010
- Lindberg K, Grozman V, Karlsson K, et al. The HILUS-Trial-a prospective Nordic multicenter phase 2 study of ultracentral lung tumors treated with stereotactic body radiotherapy. J Thorac Oncol. April 2021:1-11. doi:10.1016/j.jtho.2021.03.019
- Phase II study of accelerated hypofractionated three-dimensional conformal radiotherapy for stage T1-3 N0 M0 non-small cell lung cancer: NCIC CTG BR.25. J Nat Cancer Inst. 2014;106(8):dju164-dju164. doi:10.1093/jnci/dju164
- Onishi H, Araki T, Shirato H, et al. Stereotactic hypofractionated high-dose irradiation for stage I nonsmall cell lung carcinoma: clinical outcomes in 245 subjects in a Japanese multiinstitutional study. Cancer. 2004;101(7):1623-1631. doi:10.1002/cncr.20539
- Sim AJ, Kaza E, Singer L, Rosenberg SA. A review of the role of MRI in diagnosis and treatment of early stage lung cancer. Clin Transl Radiat Oncol. 2020;24:16-22. doi:10.1016/j.ctro.2020.06.002
- Sibolt P, Andersson LM, Calmels L, et al. Clinical implementation of artificial intelligence-driven cone-beam computed tomography-guided online adaptive radiotherapy in the pelvic region. Phys Imag Radiat Oncol. 2021;17:1-7. doi:10.1016/j.phro.2020.12.004
- Kong F-MS, Ritter T, Quint DJ, et al. Consideration of dose limits for organs at risk of thoracic radiotherapy: atlas for lung, proximal bronchial tree, esophagus, spinal cord, ribs, and brachial plexus. Int J Radiat Oncol Biol Phys. 2011;81(5):1442-1457. doi:10.1016/j.ijrobp.2010.07.1977
- Green OL, Henke LE, Hugo GD. Practical clinical workflows for online and offline adaptive radiation therapy. Sem Radiat Oncol. 2019;29(3):219-227. doi:10.1016/j.semra
- donc.2019.02.004.
- Boyle PJ, Huynh E, Boyle S, et al. Use of a healthy volunteer imaging program to optimize clinical implementation of stereotactic MR-guided adaptive radiotherapy. Tech Innov Patient Support Radiat Oncol. 2020;16:70-76. doi:10.1016/j.tipsro.2020.10.004
- Alongi F, Rigo M, Figlia V, et al. 1.5 T MR-guided and daily adapted SBRT for prostate cancer: feasibility, preliminary clinical tolerability, quality of life and patient-reported outcomes during treatment. March 2020:1-9. doi:10.1186/s13014-020-01510-w
- Chuong MD, Bryant J, Mittauer KE, et al. Ablative 5-fraction stereotactic magnetic resonance-guided radiation therapy with on-table adaptive replanning and elective nodal irradiation for inoperable pancreas cancer. Pract Radiat Oncol. 2021;11(2):134-147. doi:10.1016/j.prro.2020.09.005
- de Mol van Otterloo SR, Christodouleas JP, Blezer ELA, et al. Patterns of care, tolerability, and safety of the first cohort of patients treated on a novel high-field mr-linac within the MOMENTUM study: initial results from a prospective multi-institutional registry. Int J Radiat Oncol Biol Phys. July 2021. doi:10.1016/j.ijrobp.2021.07.003
- Crockett CB, Samson P, Chuter R, in MDF, 2021. Initial clinical experience of MR-guided radiotherapy (MRgRT) for non-small cell lung cancer (NSCLC). Front Oncol. 2021;11. doi:10.3389/fonc.2021.617681/full
- Corradini S, Alongi F, Andratschke N, et al. ESTRO-ACROP recommendations on the clinical implementation of hybrid MR-linac systems in radiation oncology. Radiother Oncol. 2021;159:146-154. doi:10.1016/j.radonc.2021.03.025
- Tocco BR, Kishan AU, in TMF, 2020. MR-guided radiotherapy for prostate cancer. Front Oncol. 2020;10. doi:10.3389/fonc.2020.616291/full
- Chin S, Eccles CL, McWilliam A, et al. Magnetic resonance-guided radiation therapy: a review. J Med Imaging Radiat Oncol. 2020;64(1):163-177. doi:10.1111/1754-9485.12968
- Kurz C, Buizza G, Landry G, et al. Medical physics challenges in clinical MR-guided radiotherapy. Radiat Oncol. 2020;15(1):93–16. doi:10.1186/s13014-020-01524-4
- Dunlap NE, Cai J, Biedermann GB, et al. Chest wall volume receiving >30 Gy predicts risk of severe pain and/or rib fracture after lung stereotactic body radiotherapy. Int J Radiat Oncol Biol Phys. 2010;76(3):796-801. doi:10.1016/j.ijrobp.2009.02.027
- Jumeau R, Filion E, Bahig H, et al. A dosimetric parameter to limit chest wall toxicity in SABR of NSCLC. BJR. 2017;90(1075):20170196. doi:10.1259/bjr.20170196
- Videtic GM, Paulus R, Singh AK, et al. Long-term follow-up on NRG oncology RTOG 0915 (NCCTG N0927): a randomized phase 2 study comparing 2 stereotactic body radiation therapy schedules for medically inoperable patients with stage I peripheral non-small cell lung cancer. Int J Radiat Oncol Biol Phys. 2019;103(5):1077-1084. doi:10.1016/j.ijrobp.2018.11.051
- Manyam BV, Videtic GMM, Verdecchia K, Reddy CA, Woody NM, Stephans KL. Effect of tumor location and dosimetric predictors for chest wall toxicity in single-fraction stereotactic body radiation therapy for stage I non-small cell lung cancer. Pract Radiat Oncol. 2019;9(2):e187-e195. doi:10.1016/j.prro.2018.11.011
- Finazzi T, Palacios MA, Haasbeek CJA, et al. Stereotactic MR-guided adaptive radiation therapy for peripheral lung tumors. Radiother Oncol. 2020;144:46-52. doi:10.1016/j.radonc.2019.10.013
- Finazzi T, van Sörnsen de Koste JR, Palacios MA, et al. Delivery of magnetic resonance-guided single-fraction stereotactic lung radiotherapy. Phys Imag Radiat Oncol. 2020;14:17-23. doi:10.1016/j.phro.2020.05.002
- Chuong MD, Kotecha R, Mehta MP, et al. Case report of visual biofeedback-driven, magnetic resonance-guided single-fraction SABR in breath hold for early stage non-small-cell lung cancer. Med Dosim. 2021;46(3):247-252. doi:10.1016/j.meddos.2021.01.003
- Finazzi T, Palacios MA, Spoelstra FOB, et al. Role of on-table plan adaptation in mr-guided ablative radiation therapy for central lung tumors. Int J Radiat Oncol Biol Phys. 2019;104(4):933-941. doi:10.1016/j.ijrobp.2019.03.035
- Lindberg K, Grozman V, Karlsson K, et al. The HILUS-Trial: a prospective Nordic multicenter phase 2 study of ultracentral lung tumors treated with stereotactic body radiotherapy. J Thorac Oncol. April 2021. doi:10.1016/j.jtho.2021.03.019.
- Pomp J, van Asselen B, Tersteeg RHA, et al. Sarcoma of the heart treated with stereotactic MR-guided online adaptive radiation therapy. Case Rep Oncol. 2021;14(1):453-458. doi:10.1159/000513623
- Shi DD, Liu KX, Hacker F, et al. Development and implementation of an online adaptive stereotactic body radiation therapy workflow for treatment of intracardiac metastasis. Pract Radiat Oncol. February 2021. doi:10.1016/j.prro.2021.01.009
- Corradini S, Bestenbostel von R, Romano A, et al. MR-guided stereotactic body radiation therapy for primary cardiac sarcomas. Radiat Oncol. 2021;16(1):60-68. doi:10.1186/s13014-021-01791-9
- Sim AJ, Palm RF, DeLozier KB, et al. MR-guided stereotactic body radiation therapy for intracardiac and pericardial metastases. Clin Transl Radiat Oncol. 2020;25:102-106. doi:10.1016/j.ctro.2020.10.006
- Mauro N, Utzeri MA, Varvarà P, Cavallaro G. Functionalization of metal and carbon nanoparticles with potential in cancer theranostics. Molecules. 2021;26(11):3085. doi:10.3390/molecules26113085
- Verry C, Dufort S, Villa J, et al. Theranostic AGuIX nanoparticles as radiosensitizer: a phase I, dose-escalation study in patients with multiple brain metastases (NANO-RAD trial). Radiother Oncol. 2021;160:159-165. doi:10.1016/j.radonc.2021.04.021
- Elguindi S, Zelefsky MJ, Jiang J, et al. Deep learning-based auto-segmentation of targets and organs-at-risk for magnetic resonance imaging only planning of prostate radiotherapy. Phys Imag Radiat Oncol. 2019;12:80-86. doi:10.1016/j.phro.2019.11.006
- Amjad A, Xu J, Thill D, et al. Deep learning-based auto-segmentation on CT and MRI for abdominal structures. Radiat Oncol Biol. 2020;108(3, Supplement):S100-S101.
- Rigaud B, Anderson BM, Yu ZH, et al. Automatic segmentation using deep learning to enable online dose optimization during adaptive radiation therapy of cervical cancer. Int J Radiat Oncol Biol Phys. 2021;109(4):1096-1110. doi:10.1016/j.ijrobp.2020.10.038
- McNair HA, Wiseman T, Joyce E, Peet B, Huddart RA. International survey; current practice in on-line adaptive radiotherapy (ART) delivered using Magnetic Resonance Image (MRI) guidance. Tech Innov Patient Support Radiat Oncol. 2020;16:1-9. doi:10.1016/j.tipsro.2020.08.002
- McNair HA, Joyce E, O’Gara G, et al. Radiographer-led online image guided adaptive radiotherapy: a qualitative investigation of the therapeutic radiographer role. Radiography. June 2021. doi:10.1016/j.radi.2021.04.012
- McIntosh C, Conroy L, Tjong MC, et al. Clinical integration of machine learning for curative-intent radiation treatment of patients with prostate cancer. Nat Med. June 2021:1-7. doi:10.1038/s41591-021-01359-w
Citation
Singer L, Kann BH, Cagney DN, Leeman JH, Yom SS, Kozono D. Actualizing Risk-Adapted Thoracic Stereotactic Body Radiation Therapy with MR Guidance . Appl Radiat Oncol. 2021;(3):6-14.
October 5, 2021