A Retrospective Validation of Patient Immobilization Using the CIVCO trUpoint ARCH With Helical Tomotherapy for Intracranial SRS
Images
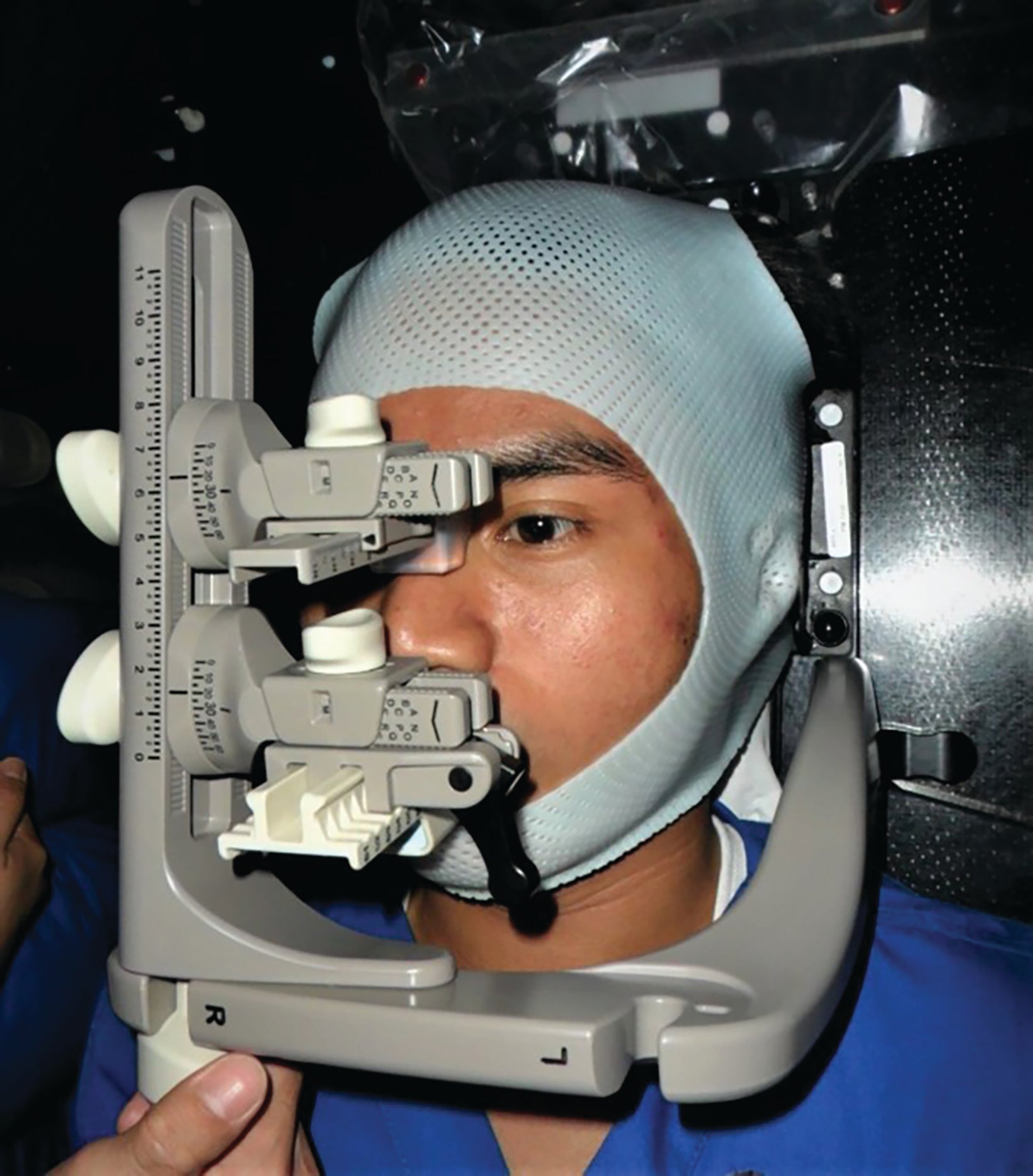
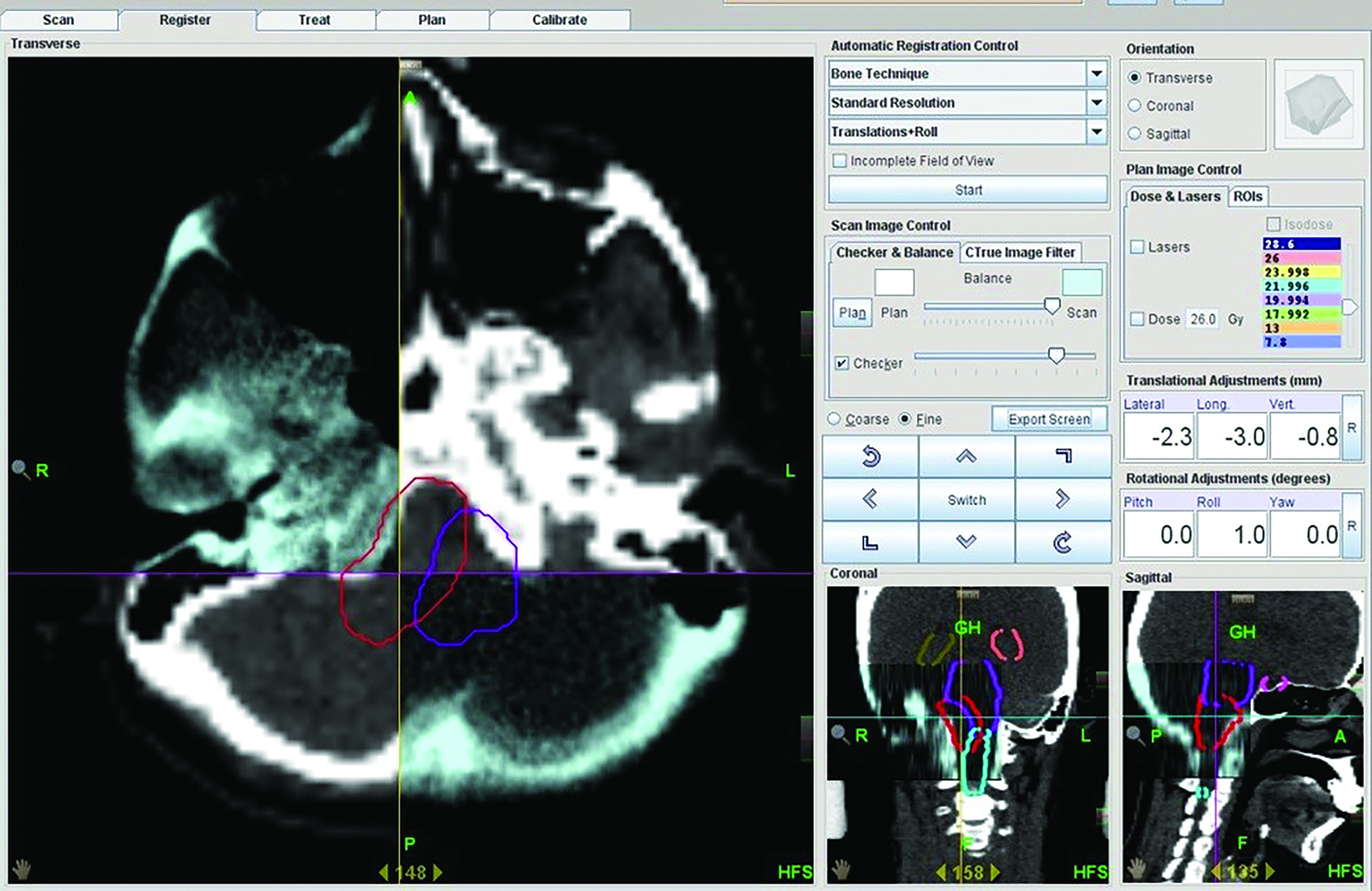
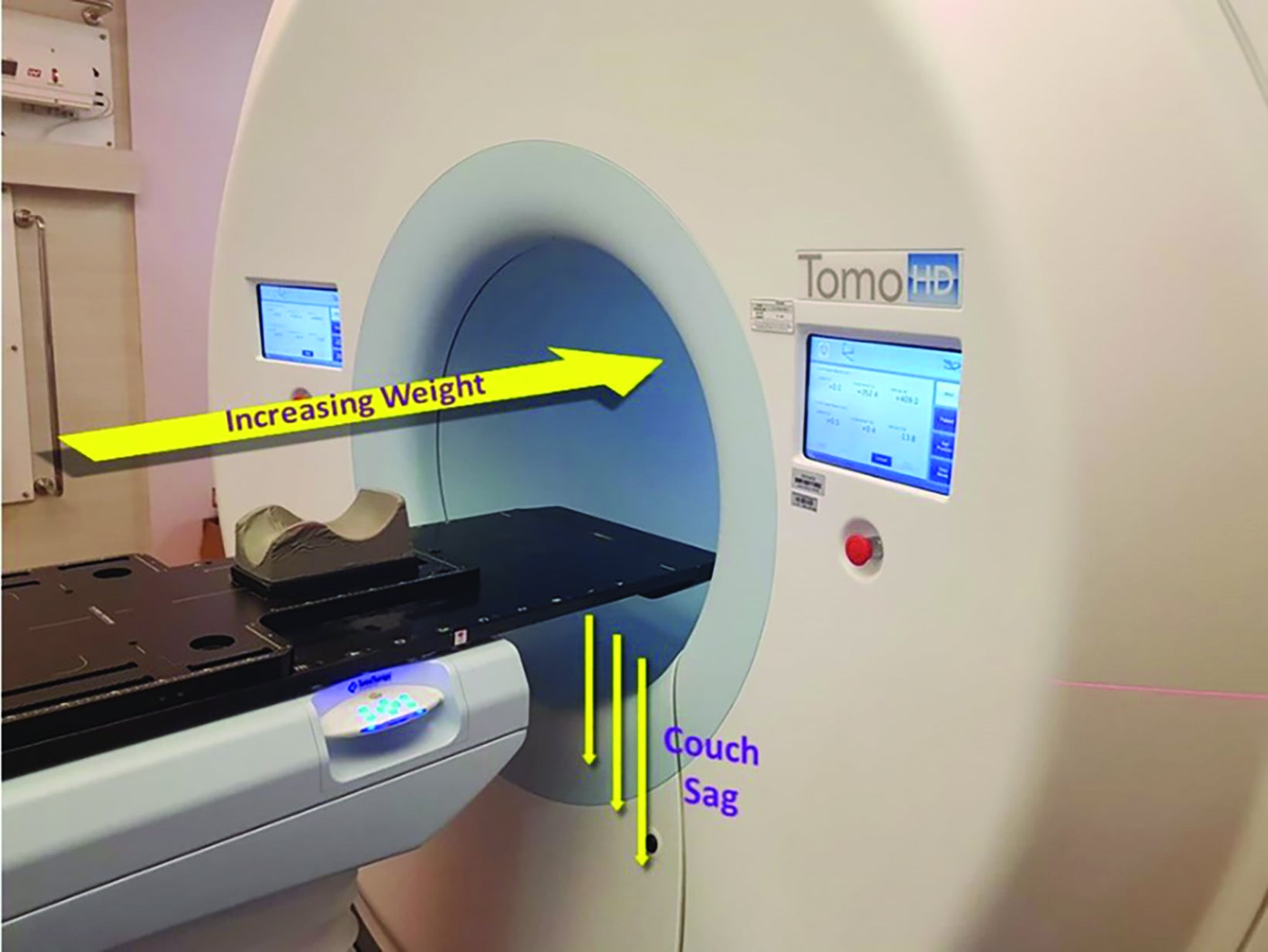
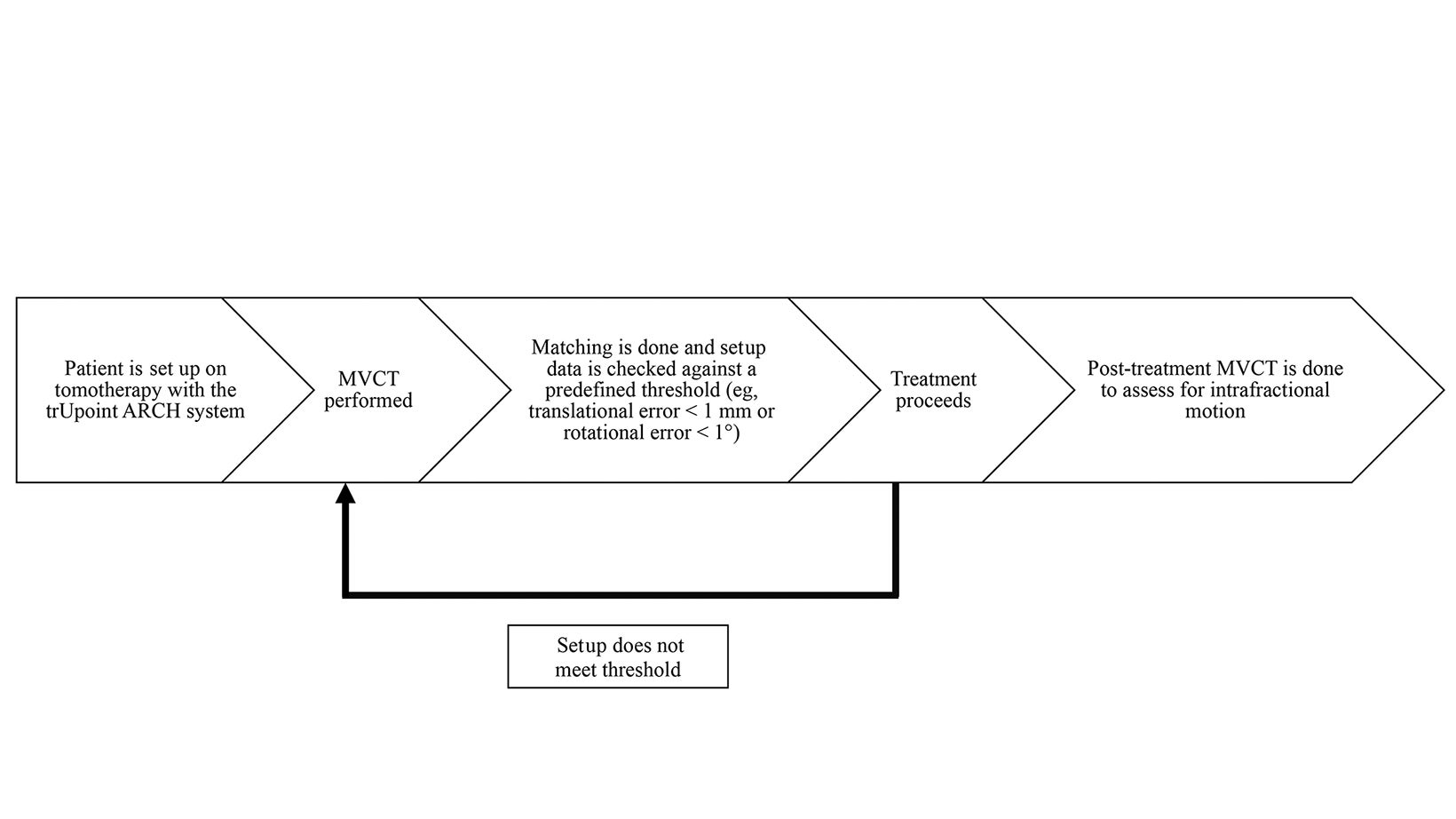
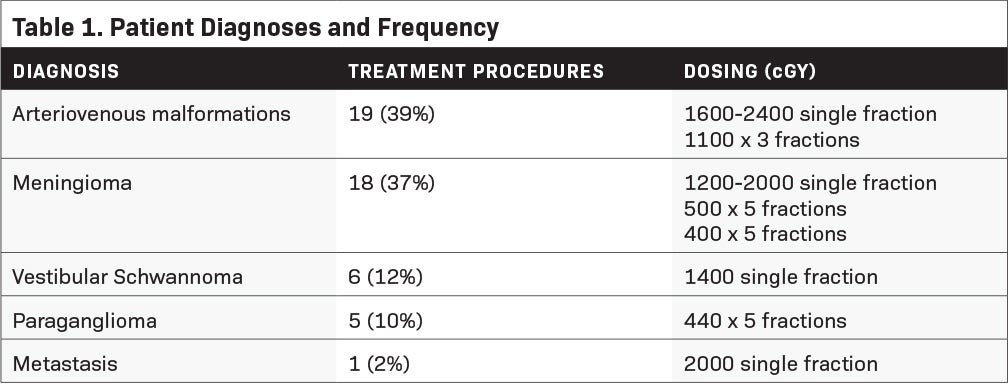
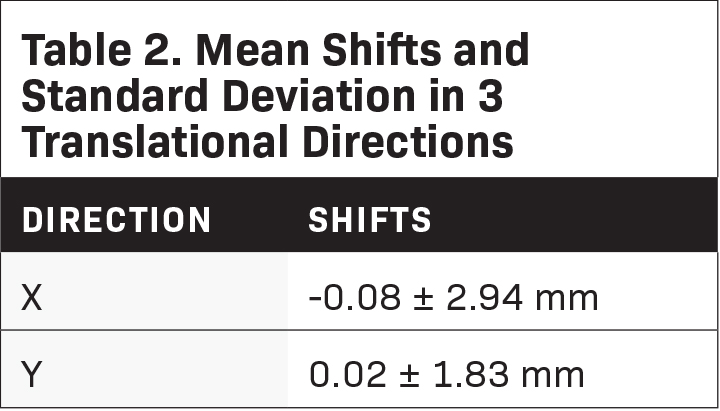
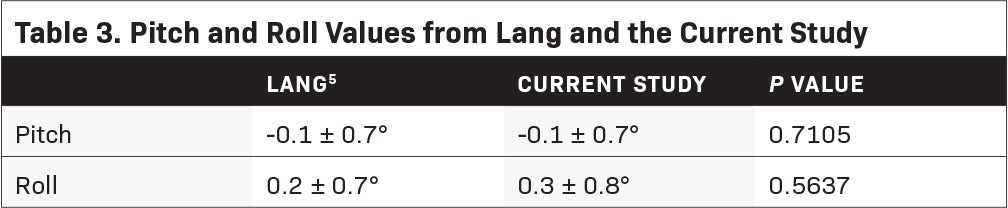
Abstract
Background: Frameless stereotactic radiosurgery and fractionated stereotactic radiation therapy (SRS/FSRT) procedures are increasingly being done. The CIVCO TrUpoint ARCH in combination with helical tomotherapy using an image-guided radiation therapy (IGRT) approach has been used in our department since January 2017. This retrospective serves as an interim performance report for the use of device in a Philippine community setting where access to frame-based SRS/FSRT is limited.
Materials and Methods: Analysis was done for patients treated from January 1, 2017, to December 31, 2018. Setup shifts (X-horizontal, Y-vertical, Z-anteroposterior), roll and pitch (rotations about the superior-inferior and mediolateral axes) measurements were obtained after pretreatment megavoltage computed tomography
(MVCTs). Planned beam-on time was recorded. The total 3-D deviation was calculated by adding the total translational corrections in quadrature.
Results: Twenty-five patients (49 stereotactic intracranial procedures) were treated. The mean couch shifts after imaging were -0.08 mm (± 2.94), 0.02 mm (± 1.83), and 4.40 mm (± 5.15) for the X, Y, and Z axes, re- spectively. The total 3-D displacement was found to be 5.68 mm (± 2.41). The obtained pitch and roll values were -0.1 degrees (± 0.7) and 0.3 degrees (± 0.8). Our rotational displacements were found to be comparable to a previous publication (P = 0.7105 for pitch and P = 0.5637 for roll). Mean beam-on time was 13.57 minutes (± 5.67).
Conclusion: The pretreatment displacements were found to be high compared to the submillimeter to approximately 4-mm shifts in previous reports. A discordance between the lasers of the CT simulator and the treatment machine was found on process review. Rotational displacements remain < 1 degree and image guidance through MVCT has allowed treatments to continue. The combination of frameless SRS/FSRT and IGRT is promising in a resource-limited setting.
Keywords: Stereotactic radiation, stereotactic radiosurgery, SRS, linac radiosurgery, linac radiosurgeries, immobilization, helical tomotherapy, image-guided radiotherapy, image-guided radiation therapy, IGRT
Conventionally, fractionated radiation therapy exploits the differential sensitivity of target tissues and normal organs. The accumulation of small daily doses damages rapidly proliferating tumors more than organs at risk (OARs). In contrast, stereotactic radiosurgery (SRS) refers to the delivery of a single, ablative, high-dose fraction of radiation, and fractionated stereotactic radiation therapy (FSRT) refers to the delivery of limited fractions of high-dose radiation. In these techniques, small targets are irradiated employing multiple non-coplanar converging beams. The radiobiologic principles of this treatment appear to be distinct from that of conventional radiation therapy. Normal tissue sparing is achieved through a steep dose fall-off.1 This modality has been used for a variety of functional, benign, and malignant intracranial conditions.2,3
Nuances in a stereotactic treatment include the high conformal doses, which can be proximal to critical structures and long treatment times, which increase the chances of intra-fractional motion. This requires accurate patient positioning to reduce geometric uncertainties. Patient immobilization is also key to reduce motion.4 Patients undergoing intracranial stereotactic radiosurgery classically have been immobilized with the use of an invasive frame bored into the patient’s skull; the first of these techniques was described by Leksell in 1950.3 Aside from fixation, these invasive frames are also meant to localize the lesion within the cranial vault, at a time when axial 3-dimensional (3-D) imaging was not in wide use. These invasive devices have been designed to keep positional shifts within ≤ 1 mm. In these conventional systems, a typical workflow consists of simulation, planning, and treatment, all compressed in 1 day.
Modern linear accelerator-based radiosurgical systems are now equipped with on-board computed tomography (CT) scanning capabilities to assist in the localization of tumors. These have virtually eliminated the need for invasive fixation. In this setting, motion is minimized with the use of an individualized mask or frame that is created for the patient prior to treatment.1 These personalized devices are increasingly being utilized in radiation therapy departments and are valued for being noninvasive, time-efficient, and simple. Patients no longer require a minor procedure to affix the cumbersome frame, and departmental resources as well as manpower are streamlined.5 This may be of importance in a developing setting where resource scarcity is magnified and access to frame-based techniques is limited.
Patients may undergo a planning procedure during which a device is tailored to the patient’s specific anatomy. They may then be discharged immediately after and radiosurgery may be done on another day, allowing adequate time to plan for optimal beam arrangements. Frameless immobilization devices also allow for the delivery of multiple high-dose treatment fractions on an outpatient basis.
Frameless immobilization has previously been evaluated. In 2015, Lang and colleagues validated the use of the trUpoint ARCH fixation system (CIVCO Medical Solutions) with stereotactic volumetric modulated arc therapy on a TrueBeam (Varian) linear accelerator. Their system yielded an average 3-D setup error of 2.1 ± 2.9 mm. The mean pitch and roll values were -0.1 ± 0.7 degrees and 0.2 ± 0.7 degrees with 98.0% and 98.9% of the pitch and roll values being < 1.5 degrees. The maximum intrafractional motion was 2.0 mm in the longitudinal direction and 95% of the total shifts were < 1.4 mm.5
In Makati Medical Center (MMC), treatments were done with the use of 2 tomotherapy (HD and Hi-ART) units that have MVCT capabilities. From January 2017 up to December 2018, a total of 49 SRS procedures on 25 patients have been done. Similar combinations of immobilization and treatment were not found on review. This retrospective analysis was carried out as an interim evaluation of the performance of the department. The results of this study have important implications for a wider adaptation and acceptance of similar treatment structures in the community, where there may be reluctance to depart from traditional frame-based systems.
Methods and Materials
Study Design
The study retrospectively analyzed all patients who were treated with SRS or FSRT in MMC from January 1, 2017 to December 31, 2018 after appropriate internal review board (IRB) evaluation and clearance, with the goal of carrying out an interim evaluation for the treatment technique. Electronic charts were searched for details of treatment. Specific data collected included setup shifts (X-horizontal, Y-vertical, Z-anteroposterior),5 roll and pitch defined as rotations about the superior-inferior and mediolateral axes, respectively,6 and treatment time defined as planned beam-on time. The total 3-D deviation was obtained by adding the total translational corrections in quadrature.7
Planning Technique
The patients were immobilized using the trUpoint ARCH fixation system. This system8 was made of multiple customizable components. These components, mounted on an arch apparatus, included the head support, bite tray, thermoplastic mask, and lower nasion cushion (Figure 1).9 A compatible baseplate was used for the attachment of the arch. Each element was customized to a patient’s specific anatomy and recorded at the time of simulation.10
A Somatom Perspective (Siemens Healthineers) was used for CT simulation. One-mm slices were used for image acquisition and contouring of targets was done on the Pinnacle3 (Philips), aided by pre-simulation images (contrast-enhanced MRI or CT scans) that were fused to the planning slices. Patients were required to have an MRI scan at most 2 weeks prior to the simulation but most had an MRI at the time of CT simulation. Patients were discharged after CT simulation, and treatment planning was done on an outpatient basis.
Patient Characteristics
Twenty-five patients, spanning 49 stereotactic intracranial procedures, were treated using the CIVCO trUpoint ARCH. The mean age of the patients was 42.62 years (19 to 85). Fourteen (56%) males and 11 (44%) females were treated. The majority of patients were treated for arteriovenous malformations (AVM) or meningiomas (Table 1). Eighteen patients (72%) received SRS while 7 patients (28%) received FSRT. Of those who received multiple fractions, 6 patients were treated on consecutive days while 1 was treated on a “split-type” protocol of 2 high-dose fractions given to different sections of the treated volume 7 months apart.
Treatment Technique
Treatment was delivered with helical image-guided intensity modulated radiation therapy (IG-IMRT) with the use of either one of two 6-MV tomotherapy linear accelerators. Prior to treatment, patients were set up on the treatment couch with the same configuration recorded at the time of simulation; 1-mm pretreatment MVCT images were acquired and auto-matched to the reference CT images obtained during simulation. Corrections were made on a 4-degree-of-freedom (4-DoF) couch (Figure 2).
Matched images were verified by attending physicians (radiation oncologist/neurosurgeon) based on bony anatomy, and in some instances, internal anatomy (eg, structural displacement, air locules, surgical clips, ventricular system) when visible on MVCT. Acquired shifts were then put on record in the patient’s chart. No repeat imaging was done for verification after corrections were made, and post-treatment MVCT was not done.
Statistical Analysis
The data was encoded using Microsoft Excel and checked for completeness, consistency, and errors. Data analysis was done through Stata 15. Descriptive statistics (mean and standard deviation) were used to evaluate the collected data while comparison of the acquired information to published data was carried out with the 1 sample z-test. P values < 0.05 were considered statistically significant.
Results
The mean couch shifts after MVCT were -0.08 mm (± 2.94), 0.02 mm (± 1.83), and 4.40 mm (± 5.15) for the X, Y, and Z axes, respectively (Table 2). The total 3-D displacement was found to be 5.68 mm (± 2.41). The obtained pitch and roll values were -0.1 degrees (± 0.7) and 0.3 degrees (± 0.8). A comparison of the obtained values was made with a previous publication from the University Hospital Zurich by Lang.5 Using the Z-test for 1 mean, mean pitch and roll values between the 2 data sets were not significantly different (Table 3). Average beam-on time was 13.57 minutes (± 5.67). In the department, treatment time was also measured from the start of patient setup to end of treatment. The overall mean treatment time was 32.9 minutes (± 10.3).
Discussion
Although frame-based radiosurgery has been the gold standard of SRS, its use has been described as a traumatic experience for patients. The procedure to affix the device involves risks such as bleeding and those associated with the use of pre-medication.
In addition, it poses a resource burden due to its requirement of dedicated nursing and physician personnel. Since treatment planning and delivery is done on the same day, advanced planning techniques like intensity-modulated radiation therapy may be harder to implement.11 A stereotactic frame also requires a rigid relationship between the device and the patient’s skull and slippage of the former has been reported, posing a risk to the fidelity of the treatment and safety of the patient.12
This study was undertaken as an interim evaluation of our performance using the trUpoint ARCH fixation system for SRS or FSRT with helical tomotherapy. The calculated total 3-D displacement was found to be large at 5.68 mm (± 2.41). The mean shifts in the X, Y, and Z axes were -0.08 mm (± 2.94), 0.02 mm (± 1.83), and 4.40 mm (± 5.15). Of note, no repeat MVCT is done after correction to confirm the patient’s position. Data from the University of Zurich show corresponding values for interfractional motion in the X, Y, and Z-axes at -0.13 mm (± 1.41), 0.04 mm (± 1.47), and -0.18 mm (± 1.03) over 248 samples.8
In Lang’s experience with the device, they determined that the average 3-D setup error was 2.1 mm (± 2.9) with pitch and roll values of -0.1 degrees (± 0.7) and 0.2 degrees (± 0.7), respectively.5 A comparison was done with our acquired values and no significant difference was found with mean pitch and roll values of -0.1 ± 0.7 (P = 0.7105) and 0.3 ± 0.8 degrees (P = 0.5637).
The mean total treatment time in Lang’s series was 10.1 minutes (± 1.4), although this was defined as the time from the first imaging procedure to the end of the second one post-treatment. In contrast, the mean beam-on time was 13.57 minutes (± 5.67), while overall treatment time in this study was 32.9 minutes (± 10.3), which included the time from when the patient was wheeled into the bunker to the end of the procedure. The difference in institutional protocols (namely, the absence of a post-treatment MVCT) and definition of treatment time was too dissimilar and a direct comparison was not made. In addition, while the tomotherapy platform relied on a fan-beam MVCT and slice-by-slice helical delivery with a monoenergetic beam, the TrueBeam linear accelerator made use of CBCT and stereotactic volumetric arc therapy.
In general, the positional reproducibility of frameless systems should vary by < 1 mm.13 The review of cases revealed that the shifts obtained within the period were substantially greater than allowed. The difference in setup accuracy was determined to be from a discordance between the lasers of the CT scan used for treatment planning and the accelerator system, and the misalignment of the lasers of the accelerator structure itself. To a lesser extent, the helical nature of treatment may result in couch sag, which could have been a potential contributor (Figure 3).
Despite the considerable translational errors, treatment proceeded as planned with image guidance from pretreatment MVCTs. With the ability to obtain volumetric image guidance, uncertainty is reduced to < 1 mm.14 No repeat imaging, however, was done to verify patient positioning before treatment was commenced. On cross-sectional imaging, the fidelity of immobilization was evident on the small rotational errors (Table 3) obtained despite the substantial translational errors, meaning the relationship of the patient’s anatomy to the immobilization system was highly conserved.
Various methods of patient frameless immobilization have been described in literature. These reports are heterogenous and differ in the kind of system (ie, thermoplastic mask, modified frame setup), the accessories used (ie, bite block, jaw support, robotic couch), and pre-treatment verification processes (ie, x-ray, CT scan). The displacements in these studies have ranged from submillimeter to approximately 4-mm shifts.
Verbakel, using a BrainLab thermoplastic mask system together with a robotic couch, determined the mean 3-D displacement on X-ray to be 0.42 mm (± 0.21) with corrections of 1.2 mm (± 0.9), 1.4 mm (± 1.3), and -0.5 mm (± 0.7) in the X, Y, and Z axes, respectively.15 The same thermoplastic mask was also evaluated by Minniti, obtaining a total 3-D displacement of 0.5 mm (± 0.7) and individual displacements of 0.5 mm (± 0.35), 0.4 mm (± 0.6), and 0.2 mm (± 0.4),16 while Theleen found a total 3-D displacement of 1.16 mm (± 0.68) and mean displacements of 0.78 mm (±1.01), 0.14 mm (± 0.37), and 0.18 mm (± 0.25).17 Santvoort modified this thermoplastic mask system using a vacuum mouthpiece or a standard upper jaw support and obtained mean 3-D average vector lengths of 1.7 mm (± 0.7) and 2.1 mm (± 1.2) for each system, respectively.18
Tryggestad et al presented their experience with 4 types of CIVCO thermoplastic masks and obtained mean interfractional shifts ranging from 2.9 mm (± 1.5) to 2.1 mm (± 1.0).19 Babic et al tested the gold-standard invasive Cosman-Roberts-Wells (CRW) frame against the relocatable Gill-Thomas-Cosman (GTC) frame, thermoplastic Uniframe, thermoplastic Orfit, and noninvasive PinPoint frame.20 Using a 6-degree-of-freedom robotic couch top, they obtained the lowest initial mean 3-D setup error with the CRW frame (0.67 mm) followed by the PinPoint system (2.06 mm and 2.15 mm for SRS and fractionated stereotactic radiation therapy). The rest of the systems yielded shifts approximately double these values.20
The performance of a thermoplastic mask was also analyzed by Masi.21 Two setups were made: one with a bite block and the other with none. The mean 3-D displacements for each of these systems were 2.9 mm (± 1.3) and 3.2 mm (± 1.5). Three systems were evaluated by Baumert: a relocatable head mask system alone or in combination with a bite-block or upper jaw support.22 Mean deviations were calculated 2.2 mm (±1.1), 3.3 mm (±1.8), and 3.7 mm (±2.8) for the bite-block, upper jaw support, or plain system, respectively.22
Because of the diverse methodologies involved in these different series, it would be difficult to make a direct comparison among them. These publications, however, put an emphasis on compensation for the loss of rigid immobilization with invasive head frames. In this case, much importance is given to the role of accurate image guidance as this will allow correction for patient movement or positional errors.
A major weakness identified in this program was the inaccuracy of the initial setup, most likely a result of the discordant laser systems. A more stringent quality assurance program would be essential to improve operations. This would entail documenting and accounting for all discrepancies (eg, simulation and treatment lasers, quantifying couch sag) and minimizing the potential consequences (eg, confirmation of corrections made before treatment, conducting post-treatment imaging).
Lee and Babic describe an ideal workflow in an image-guided, frameless setup.13 In this retrospective review, no further verification was done to check for the accuracy of the setup after the initial MVCT and positional corrections. This would have confirmed that the discordance between the laser systems of the CT simulator and tomotherapy units has been accounted for. In addition, post-treatment MVCTs were not done, which could have provided surrogate data on intrafractional motion (Figure 4). A limitation of the department was the inability to correct with 6 degrees of freedom, an intervention that helps achieve submillimeter accuracy. Adjustments in pitch and roll had to be done manually, precluding fine correction, which may introduce further displacements and lengthening overall treatment times. Finally, clinical outcomes have also not been addressed in this study and would be a suitable area of investigation. Most patients in this retrospective analysis were treated for benign intracranial conditions. Control rates may be an area of interest in future follow-up.
This study, however, provides proof of concept that a program of frameless SRS/FSRT combined with IGRT is feasible and allows for sufficient clinician confidence in treating a variety of intracranial lesions. This is foreseen to be adaptable in radiation oncology departments in a country burdened with resource scarcity and an archipelagic geography, factors that hinder patient access to adequate treatment.
Conclusion
In this interim evaluation of the institution’s 2-year SRS/FSRT program, the pretreatment displacements were found to be high with a mean 3-D vector of 5.68 mm (± 2.41) in comparison to available papers that have published submillimeter to approximately 4-mm shifts. This has been attributed to a discordance between the lasers of the CT simulator and the treatment units, a discovery made through this study. At the time of writing, all data were gathered prior to correction of this mismatch.
Despite these findings, rotational displacements remain < 1 degree. Accurate volumetric image guidance through MVCT has allowed treatment to continue despite these limitations. Repeat MVCTs after positional corrections (to confirm positioning) and post-treatment (as a surrogate to detect intrafractional motion) should be done. This study shows, however, that a combination of frameless SRS/FSRT in combination with IGRT is a promising technique in a resource-limited setting. It is a relatively simple setup and is easily adaptable to other facilities.
References
- Chen CC, Chapman PH, Loeffler JS. Stereotactic cranial radiosurgery. Published online March 9, 2018. Accessed October 22, 2018. https://www.uptodate.com/ contents/stereotactic-cranial-radiosurgery/print
- Flickinger JC. Stereotactic radiosurgery, In: Perez CA, Brady LW, Halperin CA, Schmidt-Ullrich RK, eds. Principles and Practice of Radiation Oncology. Lippin- cott Williams & Wilkins; 2018.
- Shell MC, Bova FJ, Larson DA, et al. Stereotactic radiosurgery. American Institute of Physics, Inc., 1995.
- Evans J. Positioning features in current immobilization systems. In 2014 AAPM Summer School, Richmond, VA, 2014.
- Lang S, Linsenmeier C, Brown ML, Cavelaars F, Tini A, et al. Implementation and validation of a new fixation system for stereotactic radiation therapy: an analysis of patient immobilization. Prac Rad Onc. 2015;5(6):e689-e695.
- Kaiser A, Schultheiss TE, Wong JYC, et al. Pitch, roll, and yaw variations in patient positioning. Int J Radiat Oncol Biol Phys. 2006;66(3):949-955.
- American Association of Physicists in Medicine. Intracranial stereotactic positioning systems: report of the American Association of Physicists in Medicine Radiation Therapy Committee Task Group No. 68. Med Physics. 2005;32(7):2380-2398.
- Civco Medical Solutions. trUpoint ARCH technical data sheet. Published online 2016. Accesssed December 22, 2018. http://civcort.com/ro/resources/Technical-Spec-Sheets/trUpointARCHDataTechSheet.pdf
- Civco Medical Solutions. trUpoint ARCH brochure. Published online 2016. Accesssed December 22, 2018. http://civcort.com/ro/resources/brochures/trUpoint-ARCH-Brochure.pdf
- Civco Medical Solutions. trUpoint ARCH setup sheet. Published online 2017. Accesssed December 22, 2018. http://civcort.com/ro/resources/setup-sheets/ SRS-Headframe-Setup-Sheet-Rev-A.pdf
- Ramakrishna N, Rosca F, Friesen S, et al. A clinical comparison of patient setup and intra-fraction motion using frame-based radiosurgery versus a frameless image-guided radiosurgery system for intracranial lesions. Radiother Oncol. 2010; 95:109-115.
- Otto K, Fallone G. Frame slippage verification in stereotactic radiosurgery. Int J Radiat Oncol Biol Phys. 1998;41(1):199-205.
- Lee Y, Babic S. From frame to frameless: brain radiosurgery. Image-guided Hypofractionated Stereotactic Radiosurgery. CRC Press; 201:118-135.
- De Los Santos J, Popple R, Agazaryan N, et al. Image guided radiation therapy (IGRT) technologies for radiation therapy locaization and delivery. Int J Radiat Oncol Biol Phys. 2013;87(1)33-45.
- Verbakel WF, Lagerwaard FJ, Verduin AJ, et al. The accuracy of frameless stereotactic intracranial radiosurgery. Radiother and Oncol. 2010;97:390-394.
- Minniti G, Scaringi C, Clarke E, et al. Frameless linac-based stereotactic radiosurgery (SRS) for brain metastases: analysis of patient repositioning using a mask fixation system and clinical outcomes. Radiat Oncol. 2011;6:158.
- Theelen A, Martens J, Bosmans G, et al. Relocatable fixation systems in intracranial stereotactic radiotherapy. Accuracy of serial CT scans and patient acceptance in randomized design. Strahlenther Onkol. 2012;188:84-90.
- Van Santvoort J, Wiggenraad R, Bos P. Positioning accuracy in stereotactic radiotherapy using a mask system with added vacuum mouth piece and stereoscopic x-ray positioning. Int J Radiat Oncol Biol Phys. 2008;72(1)261-267.
- Tryggestad E, Christian M, Ford E, et al. Inter- and intrafraction patient positioning uncertainties for intracranial radiotherapy: a study of four frameless thermoplastic mask-based immobilization strategies using daily cone-beam CT. Int J Radiat Oncol Biol Phys. 2011;80(1):281-290.
- Babic S, Lee Y, Ruschin M, et al. To frame or not to frame? Cone-beam CT-based analysis of head immobilization devices specific to linac-based stereotactic radiosurgery and radiotherapy. J Appl Clin Med Phys. 2018;19(2):111-120.
- Masi L, Casamassima F, Polli C, et al. Cone beam CT image guidance for intracranial stereotactic treatments: comparison with a frame guided set-up. Int J Radiat Oncol Biol Phys. 2018;71(3) 926-933.
- Baumert BG, Egli P, Studer S, Dehing C, Davis JB. Repositioning accuracy of fractionated stereotactic irradiation: assessment of isocentre alignment for different dental fixations by using sequential CT scanning. Int J Radiat Oncol Biol Phys. 2005;74:61-66.
Citation
Abrina JPF, Baldivia KH, Doromal DV. A Retrospective Validation of Patient Immobilization Using the CIVCO trUpoint ARCH With Helical Tomotherapy for Intracranial SRS. Appl Radiat Oncol. 2022;(2):23-29.
July 15, 2022